The scale of the challenge to achieve net zero is so huge that we need all the best ideas. For hard-to-abate industry executives in the audience, you’re probably looking at energy efficiency as well as alternative fuels. But you’ll still have CO₂ in your process. That’s why we believe carbon capture is a necessary piece of the decarbonization puzzle and CycloneCC, our fully modular technology, will make carbon capture simple, afforable, and scalable.
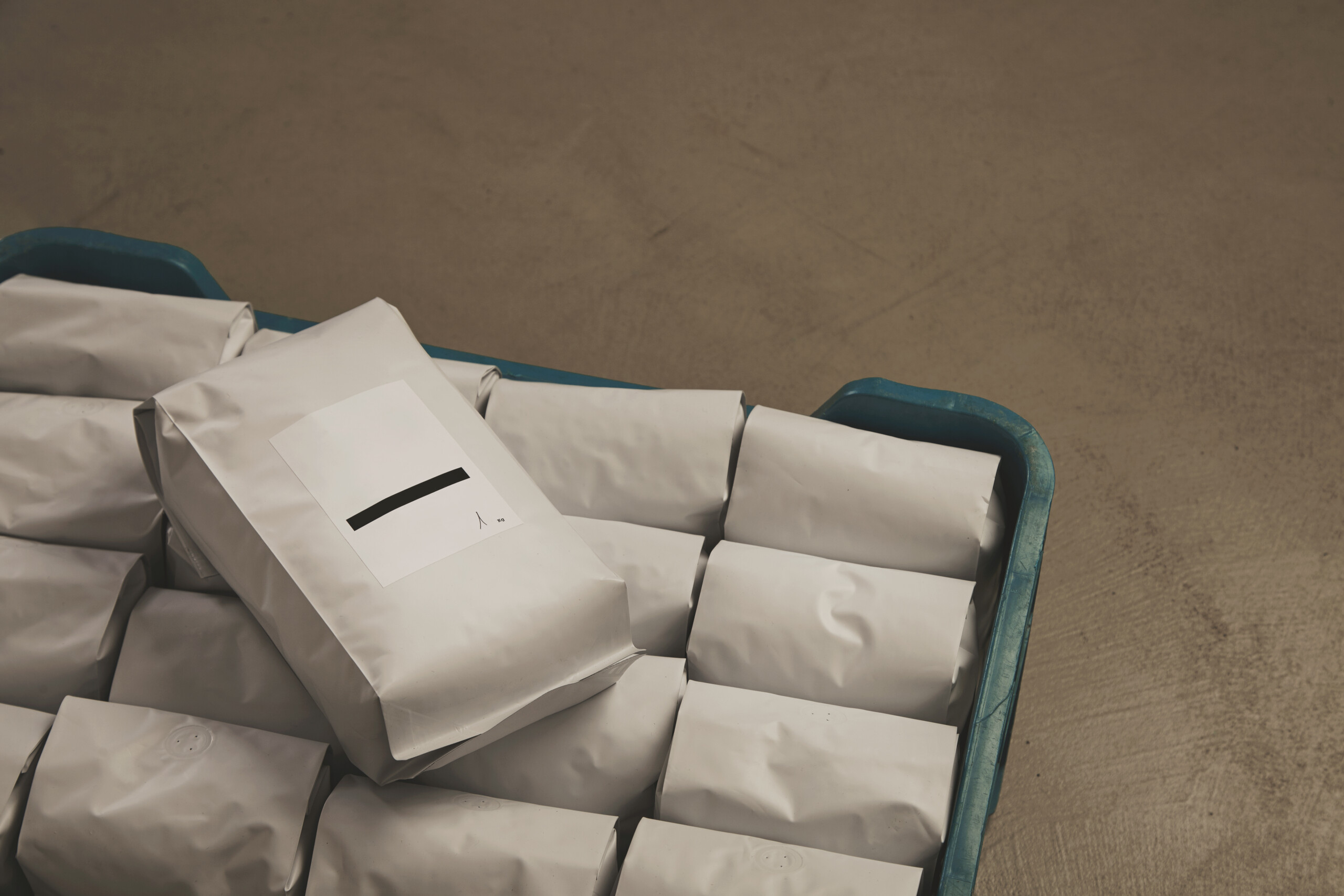
France’s Extended Producer Responsibility (EPR) framework, based on the “polluter-pays” principle, holds companies accountable for the entire life cycle of these products, from design to end-of-life.
While EPR has existed in France since 1975, it gained traction in 1992 with the household packaging decree and has since expanded to include sectors such as batteries, paper, and electrical and electronic equipment.
The major new development for 2025 is the expansion of this system to include industrial and commercial packaging (Emballages Industriels et Commerciaux – EIC). This marks a significant regulatory evolution, applying the “polluter-pays” principle to B2B packaging waste for the first time. The goal is to improve waste collection, sorting, and recycling while encouraging producers to reduce material use and invest in sustainable alternatives.
What types of packaging are affected?
The scoping study for the upcoming EPR scheme highlights that professional packaging is used across all business sectors:
- Chemical and pharmaceutical
- Cosmetics and personal care
- Manufacturing
- Textile
- Automotive
- Construction
- Transport and logistics
- E-commerce
Professional packaging includes all material used in a B2B context, including:
- Sales packaging: cans, sachets, big bags, metal drums, etc.
- Grouping packaging: cardboard boxes, plastic wrap, etc.
- Transport packaging: pallets, crates, etc.
This new regulatory framework goes beyond waste management. It is already influencing internal strategic decisions across affected industries. The obligation to manage and finance end-of-life packaging will necessarily drive companies to reconsider their materials usage, explore circular models, and implement innovative packaging solutions.
Before considering reuse, recycling or new materials, the top priority remains sobriety (i.e. reducing packaging at the source). Minimizing packaging volume and eliminating unnecessary materials is the most effective way to reduce environmental impact, and often the most cost-efficient.
Key solutions for a circular packaging system
- Reusable packaging
One of the most impactful solutions lies in reusable packaging systems. These systems drastically reduce demand for raw materials and eliminate single-use packaging by encouraging reuse cycles.
As an example: Loop partners with major brands like Nestlé to distribute products in durable, reusable containers. Once used, the packaging is collected, cleaned, and refilled—minimizing waste and optimizing material use.
- Recycled materials and bioplastics
Switching to recycled or bio-based materials can significantly reduce the environmental burden of packaging. These alternatives, often recyclable themselves, support circularity and reduce reliance on fossil-based plastics.
French startup Carbios is pioneering enzymatic recycling, a breakthrough technology that breaks down PET plastics into their base components for infinite reuse. This innovation could redefine how industries handle plastic waste.
- Packaging management tools
Smart tools can help companies monitor, reduce, and optimize their packaging usage. Digital platforms and traceability systems ensure compliance with evolving EPR regulations while making sustainability efforts more transparent.
Blockchain, for instance, can facilitate the tracking of reusable packaging throughout its life cycle, allowing companies to verify returns and reward responsible practices.
- Modular packaging
Modular packaging systems enable businesses to create packaging that fits products precisely—reducing excess material, cutting storage costs, and streamlining transport.
Packsize offers on-demand packaging solutions, allowing companies to custom-size boxes for each shipment. E-commerce players like Amazon already use this approach to reduce void fill and improve shipping efficiency.
- Natural materials
Packaging made from natural plant-based fibers—such as straw or hemp—offers a renewable, biodegradable alternative to conventional materials.
Startups like Xampla, develop plant-based materials that can replace single-use plastics. Their products, derived from pea protein, are biodegradable and suitable for various industries.
Looking ahead
As the new EPR law reshapes the industrial packaging landscape, companies are being called to adapt—not only to comply, but to lead. Circular packaging solutions like reusable systems, bio-based materials, smart management tools, and precision-fit packaging are already demonstrating how innovation and regulation can go hand in hand.
By embracing these sustainable alternatives, businesses can reduce their environmental footprint while building more resilient, future-ready supply chains.
2 Key Figures
7.5 million
tons of professional packaging were placed on the French market in 2024
7%
the rate of re-use of professional packaging in France
3 startups to draw inspiration from
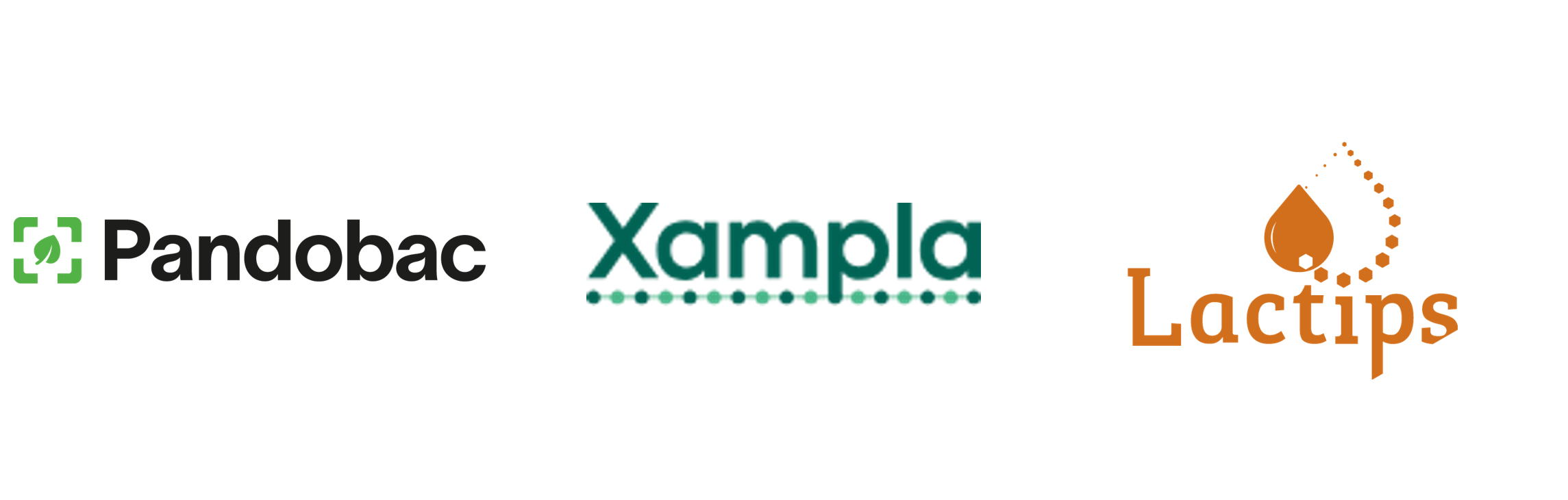
Pandobac
This French startup offers a turnkey service for the rental, tracking, and washing of reusable transport crates. The solution is tailored for B2B flows between food industry players—whether between manufacturers, retailers, or out-of-home catering providers. It enables companies to reduce single-use packaging while simplifying reverse logistics.
Xampla
A british startup that, manufactures plant-based, biodegradable packaging materials. Its product range includes stretch films, wrapping papers, and microcapsules—suitable for applications from homecare and cosmetics to packaging.
Lactips
Lactips produces water-soluble and biodegradable thermoplastic pellets using casein, a milk protein. The pellets can be used to make all sorts of packaging material.
Interested in a startup landscape or in an insights report?
Please fill out our contact form so that we can get back to you very quickly with our product offer.
Want to subscribe to our 123Fab?
Fill out our form to receive the latest insights into your inbox.
123Fab #101
1 topic, 2 key figures, 3 startups to draw inspiration from

Catalysts are substances that increase the rate of a chemical reaction without being consumed in the process. They are the unsung heroes of the industrial world and have become indispensable in a wide range of industries: chemical, pharmaceutical, petrochemical, automotive, etc. One of the best-known applications is the catalytic converter in motor vehicles, which transforms toxic gases into less harmful pollutants.
Today, they play an important role in decarbonization, with a wide range of applications, including the following:
- Production of renewable fuels: Catalysts enable the conversion of renewable raw materials, such as vegetable oils or organic waste, into sustainable fuels such as biodiesel or bioethanol.
- Green hydrogen: Catalysts are at the heart of green hydrogen production through water electrolysis. Until today, the most applied catalysts in the production of green hydrogen are noble metals such as platinum and iridium, but research is in process to find cheaper and more sustainable alternatives
- Biomass conversion: Biomass can be valorized into useful chemicals and fuels via catalysts, hence reducing further the dependance on fossil feedstock and lowering the carbon footprint of the chemical industry.
- Plastic recycling: Catalysts play an important role in chemical plastic recycling. They offer a way for the depolymerization of the polymer into its basic monomers, which can then be reused to manufacture new plastics. A good example is the enzymatic process for the recycling of PET developed by the French company Carbios.
- CO₂ conversion and utilization: Advanced catalytic processes are being developed to convert CO₂ into valuable products like fuels, polymers, and chemicals. These innovative reactions could turn carbon emissions into a raw material, and then close the carbon use loop.
However, while catalysts provide considerable environmental benefits in use, many have resource-intensive and polluting manufacturing processes. As an example, mining and processing precious metals, widely used in catalytic converters, like platinum or palladium, are often associated with ecological damage, including habitat destruction and water contamination. It is therefore crucial to find and develop new, more sustainable alternatives.
Emergence of new catalysts with lower environmental impact
To meet sustainability requirements, companies in the chemical industry are actively seeking catalytic solutions with lower environmental impact. For instance, BASF has established a Catalysts Innovations Platform dedicated to identifying more sustainable catalysts. In this context, various approaches and types of catalysts are emerging, each offering specific advantages to help achieve the industry’s sustainability goals:
- Biocatalysts, sometimes referred to as “nature’s catalysts “or enzymes are intrinsically sustainable. Their use allows to produce organic molecules in a milder manner compared to traditional chemical methods. For instance, Novozymes offers biocatalysts for biofuel production. Additionally, Solvay utilizes biocatalysts in the manufacturing of some of its polymers.
- Nanocatalysts offer several benefits compared to their traditional counterparts: the nanometric dimension offers a much better structure-performance ratio, which increases their catalytic activity and selectivity, and reduces energy consumption and the cost of chemical processes. Gen-Hy is a start-up developing catalysts based on nickel nanoparticles.
- Catalysts with abundant metals: Research is moving towards the use of more abundant and less expensive metals, such as iron, to replace precious metals like platinum. Gen-Hy, for instance, develops high-performance catalysts based on nickel nanoparticles, an abundant and inexpensive metal, to replace platinum and iridium in certain applications.
- Photocatalysts are catalysts that accelerate chemical reactions by absorbing light. This technique can be applied in interesting ways in the context of energy transition, particularly for the chemical trapping of CO₂. Researchers from the Institut lumière matière in Lyon and the Institut des sciences chimiques de Rennes are working on molybdenum aggregates, an abundant and inexpensive metal, as an alternative to noble metal-based photocatalysts.
- Ecocatalysts are quite new in sustainable chemistry. They are derived from plants that have naturally accumulated metals present in their environment during the phytoremediation process. Bioinspir has developed, for instance, ecocatalysts derived from plants for the responsible synthesis of cosmetic and perfumery ingredients.
2 Key Figures
6%
The chemical sector is responsible for approximately 6% of global CO2-equivalent emissions
$22.30 billion
The global industrial catalyst market size was nearly $22.30 billion in 2023
3 startups to draw inspiration from
Gen-Hy
A French start-up specializing in green hydrogen production has developed high-performance catalysts free of noble metals. Using an innovative formulation based on nickel nanoparticles—an abundant and affordable metal—it offers a new path toward more sustainable and cost-effective hydrogen production.
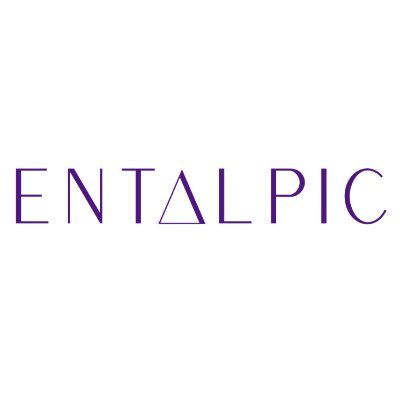
Entalpic
Founded in 2024, Entalpic is a French startup at the forefront of generative AI technology for the chemical industry. The company’s advanced AI platform designs catalysts to optimize chemical processes in areas like energy storage, fertilizer production, and pollution control, blending open and proprietary research.

Cascade Biocatalysts
Denver-based startup Cascade, specializing in enzyme-based processes, uses its Body Armor for Enzymes™ technology to drive greener, cost-effective chemical reactions that reduce greenhouse gas emissions. Its projects encompass diverse areas, including CO₂ capture, fragrance production, and wastewater treatment, highlighting the broad commercial potential of biocatalysts.
Interested in a startup landscape or in an insights report?
Please fill out our contact form so that we can get back to you very quickly with our product offer.
Want to subscribe to our 123Fab?
Fill out our form to receive the latest insights into your inbox.
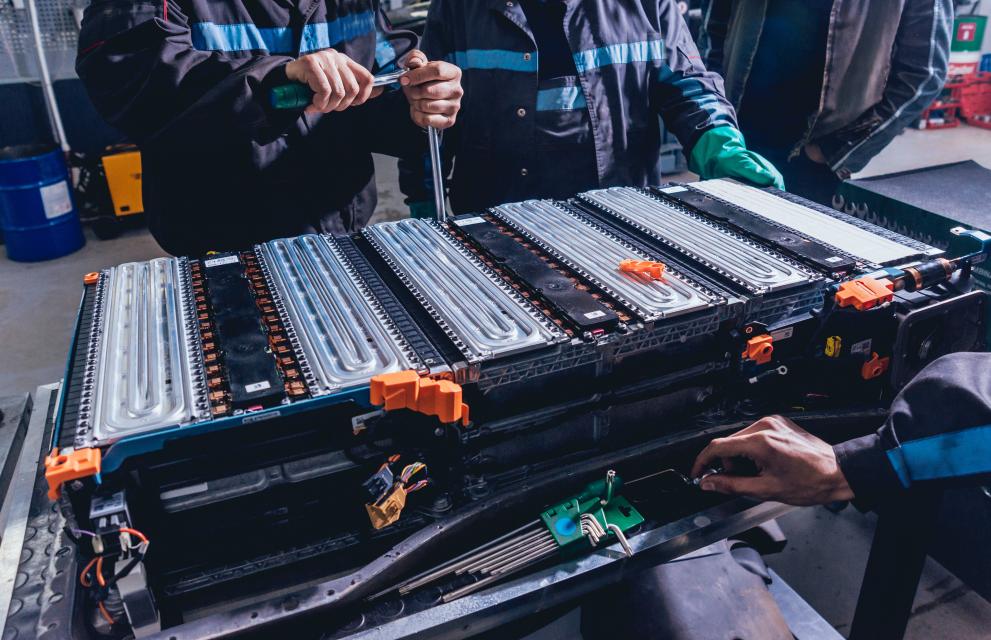
Context
Our client has developed a strong innovation activity to fuel its transformation. In this context, our client asked us to explore market demand for a new offering in dismantling and extracting valuable components from lithium-ion batteries.
Mission
We carried out a study to define the go-to-market strategy:
- Map of the second-life battery value chain, from manufacturing to end-of-life
- Segmentation of second-life component buyers
- Real-world applications of second-life components
- Interviews with 8 potential customers on interest and technical needs
- List of existing technical standards for selling second-life battery cells
- Review of the EU battery regulation compliance
- Recommendations on the go-to-market strategy and prioritization of potential customers to address first
Key figures
27
Key players identified
4
Target customer groups
8
Interviews conducted
1
Go-to-market strategy defined
123Fab #100
1 topic, 2 key figures, 3 startups to draw inspiration from
In today’s rapidly evolving agricultural landscape, regenerative agriculture has emerged as a transformative approach to address the pressing challenges of climate change, soil degradation, and biodiversity loss. This innovative methodology, first introduced in 1983 by Robert Rodale, offers concrete solutions to restore ecosystems and ensure the long-term viability of farming.
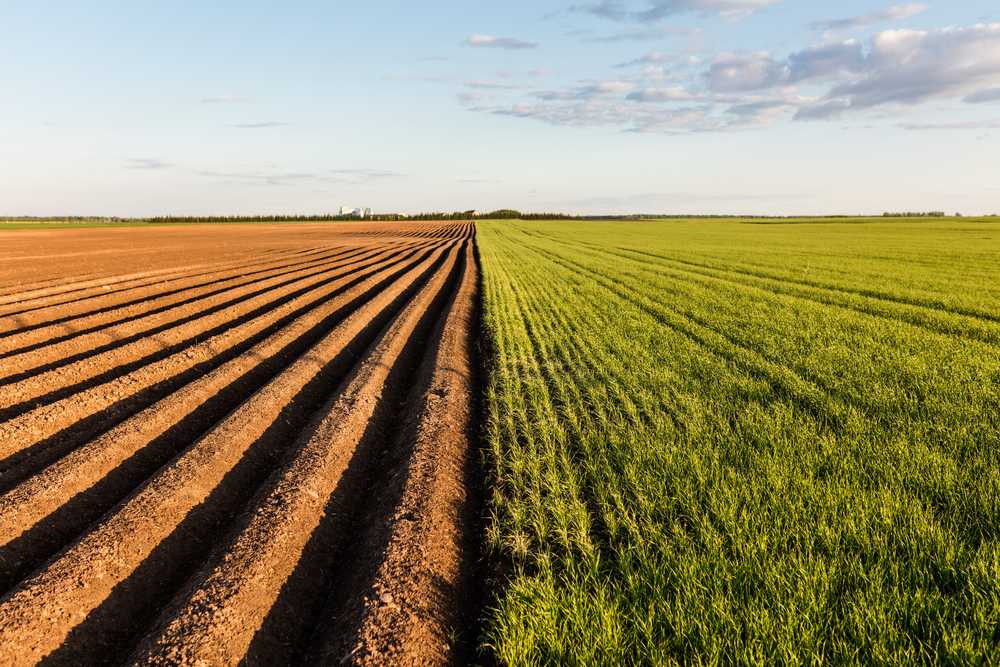
What is Regenerative Agriculture?
Regenerative agriculture is a holistic approach designed to regenerate critical resources such as soil, water, air, and biodiversity while boosting farmers’ incomes. By using regenerative farming methods, farmers can restore the natural balance of their ecosystems, leading to healthier soil, lower CO₂ emissions and increased resilience against extreme weather events like droughts and floods.
Key solutions in Regenerative Agriculture
Soil Regeneration
Soil health is the foundation of regenerative agriculture. While traditional methods like crop rotation, cover cropping, and reduced tillage remain effective, innovative techniques are emerging to further improve soil fertility:
- Precision agriculture: data-driven tools optimize farming practices. For instance, Assolia enables farmers to fine-tune crop rotations and soil management based on real-time insights into soil conditions.
- Biochar application: this form of charcoal produced by heating organic material in a low-oxygen environment, enhances soil quality, improves water retention and contributes to carbon sequestration. Companies such as NetZero and Poas Bioenergy manufacture it.
- Microbial solutions: biostimulants and biofertilizers from companies such as Veragow and Mycophyto offer natural alternatives to synthetic fertilizers, promoting soil fertility and plant health.
Water Regeneration
Water is a precious resource, yet is often taken for granted. Regenerative agriculture emphasizes the importance of efficient water use and regeneration through:
- Optimized irrigation: ensures crops receive the exact amount of water needed, reducing waste. For example, Unilever has implemented this in its Spanish tomato supply chain using soil moisture sensors, weather stations and data analytics software production to provide accurate data on water requirements.
- Water recycling: involves collecting, treating, and reusing water on the farm, through techniques such as simple filtration, artificial wetlands (which naturally filter and treat wastewater), and reverse osmosis, an advanced filtration method that removes contaminants for safe reuse
Air Quality
Regenerative agricultural practices offer a dual benefit: they significantly reduce greenhouse gas emissions—addressing the 20% of global emissions attributed to conventional agriculture (see our blogpost for a detailed breakdown of emissions by sector here)—while simultaneously enhancing the soil’s capacity to sequester carbon.
- Reduced use of chemical inputs: by minimizing reliance on synthetic fertilizers and pesticides, farmers can lower their greenhouse gas emissions.
- Reduced use of heavy machinery: regenerative practices also limit the need for heavy machinery, resulting in lower fuel consumption and reduced emissions. This not only decreases greenhouse gas output but also lowers operational costs and machinery wear.
- Carbon sequestration: techniques like no-till farming and agroforestry—planting trees and shrubs alongside crops—enhance the soil’s capacity to store carbon, effectively removing CO₂ from the atmosphere.
Farmers can also generate additional income by selling carbon credits, for the additional carbon stored in their soil, through platforms like Klim or Soil Capital, which support them in their transition to regenerative practices.
Biodiversity Enhancement
- Biodiversity monitoring: companies such as Nature Metrics utilize eDNA technology to detect individual species from small samples of soil, sediment, water, or air. This approach enables accurate monitoring of changes in both above-ground and below-ground biodiversity, including soil bacteria and fungi.
- Pest management: biological pest control, often referred to as biocontrol, is a method of managing pests using natural predators, parasitoids, or pathogens. French startup Agriodor uses natural scents emitted by plants to repel crop-destroying insects.
How can the transition to regenerative agriculture be accelerated?
The shift to regenerative agriculture can’t be left to farmers alone. Initial costs and associated risks require collaboration between farmers, governments, and the private sector. Policymakers play a crucial role by rethinking existing farming policies, providing financial incentives and offering technical support.
The private sector also has a vested interest in financing the transition to regenerative practices, as it improves their carbon footprint and enhances supply chain resilience. Many companies are already making commitments; for instance, Nestlé, the world’s largest food and beverage company, aims to source 50% of its key ingredients through regenerative agriculture by 2030.
2 Key Figures
40%
of the world’s soils are moderately to highly degraded due to conventional agriculture
$2.2 billion
the amount Danone, Pepsico, Nestlé and Cargill commited to investing in regenerative agriculture at COP28
3 startups to draw inspiration from

Klim
Headquartered in Berlin, Klim enables farmers to transition to regenerative agriculture at scale by providing financial support, knowledge, documentation tools, and a community via their digital companion for farmers. Klim-verified carbon removal credits, generated by Klim farmers, help companies offset their carbon emissions locally with maximum impact and transparency.
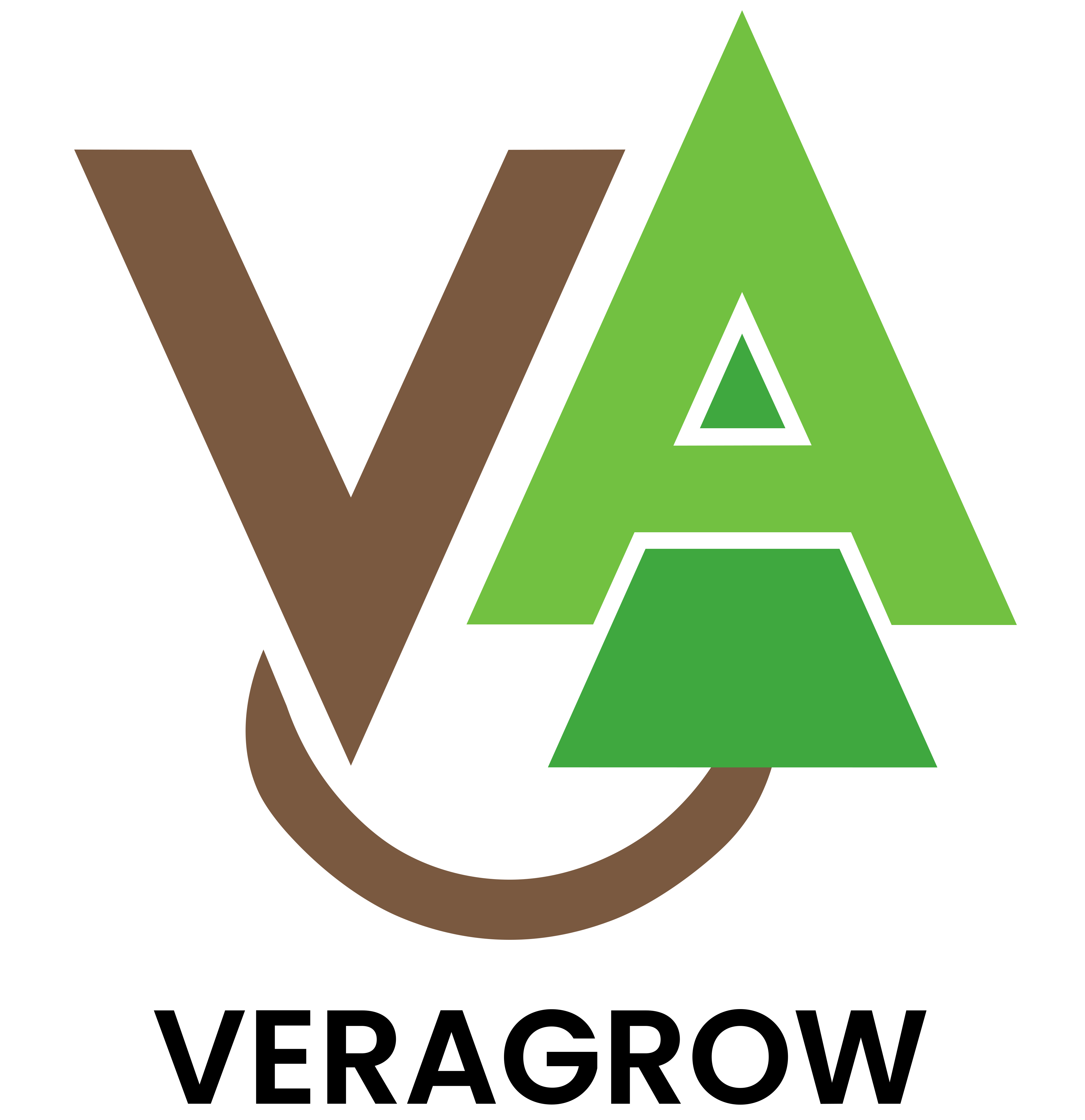
Veragrow
A French company that offers organic fertilizers made from vermicompost, i.e. earthworm droppings containing nutrients and micro-organisms beneficial to plants. An ecological alternative to pesticides that also revitalizes the soil.

Agrovar
A Bulgarian company which has developed a software specialized in precision agriculture, offering advanced tools for informed decision-making, as well as real-time crop monitoring and management. Its soil health assessment algorithms analyze essential soil data, contributing to climate resilience by helping farmers adapt to changing weather conditions.
Interested in a startup landscape or in an insights report?
Please fill out our contact form so that we can get back to you very quickly with our product offer.
Want to subscribe to our 123Fab?
Fill out our form to receive the latest insights into your inbox.
123Fab #99
1 topic, 2 key figures, 3 startups to draw inspiration from
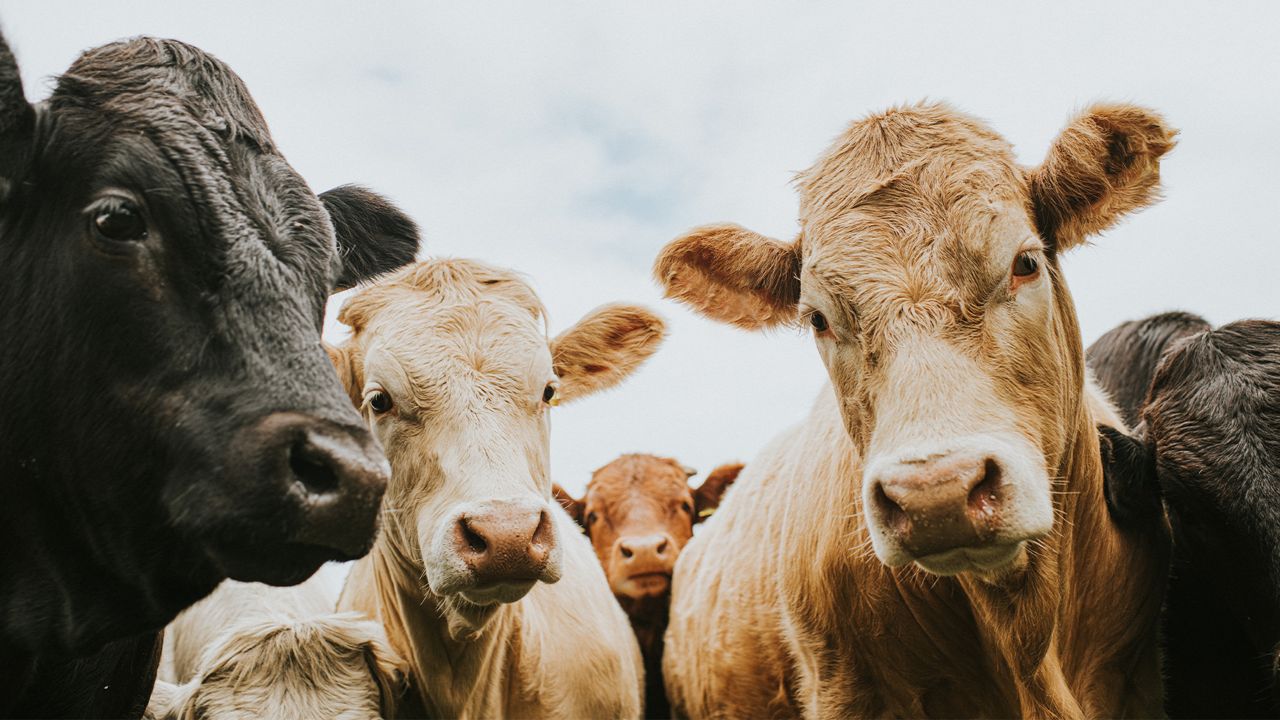
People generally link global warming with carbon dioxide (CO2) but, as the Intergovernmental Panel on Climate Change (IPCC) explains, 30% of the increase in global temperature since pre-industrial levels is due to higher methane (CH4) concentrations in the atmosphere. This is because methane is extremely more effective at trapping heat.
Where does methane come from?
The IEA has estimated that 40% of methane comes from natural sources (wetlands, biomass burning…), and the remaining 60% from human activities (agriculture, oil & gas production, waste). The two pathways to methane production are:
- Gas leaks – methane is the main component of natural gas. Thus, it can leak from pipelines and drilling.
- Decomposition of organic matter – when organic matter is in oxygen-free environments, particular microbes called methanogens take the lead in breaking down the organisms. This process, called methanogenesis, leads to the creation of methane.
According to McKinsey, five industries could reduce global annual methane emissions by 20% by 2030 and 46% by 2050. Those are agriculture, oil and gas, coal mining, solid-waste management, and wastewater management.
What about methane capture from the air?
Methane is 200 times less abundant in the atmosphere than CO2 — a scarcity that makes removing it a technical challenge. Capturing methane would require processing a lot of air, which could require an extremely large amount of energy. And unlike CO2, which can be captured both physically and chemically in a variety of solvents and porous solids, methane is completely non-polar and interacts very weakly with most materials. However, researchers claim to have found a promising solution. A class of crystalline materials, called zeolites, capable of soaking up the gas. Regardless of this solution, the difficulty of capturing methane from the air is the reason why most technologies focus on oxidizing the greenhouse gas rather than “hooking” it out.
Startups are developing innovations to curb methane emissions
For the decomposition of organic matter:
- in the gut of ruminants (like cows and cattle) – Australian startup Rumin8 and Swedish startup Volta Greentech are fighting this issue by developing seaweed-based nutritional supplements that inhibit methane production.
- on landfills and wastewater – US startup LoCi Controls bolsters the methane capture process using solar-powered devices.
- on wetlands – UK methane capture startup bluemethane has developed a technology to capture methane from water, enabling to mitigate the methane production from rice cultivation.
For gas leaks:
- oil & gas production – UK startup Kuva Systems uses short-wave infrared cameras to autonomously monitor and alert oil and gas companies about methane leaks. Whereas US startup BioSqueeze has developed a biomineralization technology that seals miniscule leakage pathways in oil and gas wells.
- melting permafrost – the trapped organic matter in the frozen seafloors or shallow seas is emitted when they thaw. US startup Blue Dot Change is investigating whether releasing ion particles into the exhaust steam of ship vessels crossing the ocean can accelerate the destruction of methane.
A methane tax just like carbon taxes
Norway was one of the first countries to introduce a carbon tax in 1991. Aside from carbon, the harmful gases regulated by the tax also include methane. All Oil & Gas operators on the country’s continental shelf are now required to report all methane emissions from their activities. As a result, studies show that the country has succeeded to consistently maintain low methane emissions. Canada is proposing to require companies to inspect their infrastructure monthly, fixing the leaks they find as part of efforts to reduce the sector’s methane emissions by 75% by 2030 (compared with 2012). Although the EU is among 150 signatories to the Global Methane Pledge – an agreement to cut emissions of methane by 30% – EU energy chief warned early March that the EU was lagging in the race to curb methane emissions. Since the proposals on methane in 2021, they have been watered down.
In short, methane will be critical to solving the net-zero equation. The good news is that mature technologies are at hand. From feed additives for cattle to new rice-farming techniques, to advanced approaches for oil and gas leak detection and landgas methane capture. Where costs are prohibitive, there is a need for coordinated action to create the infrastructure and fiscal conditions that would support further action. Finally, across the board, there is a need for more monitoring and implementation.
2 Key Figures
Budget of $60-110 billion annually up to 2030
Full deployment of the methane abatement measures would cost an estimated $150-$220 billion annually by 2040 and $230-$340 billion annually by 2050.
< 100 funded companies
Tracxn
3 startups to draw inspiration from
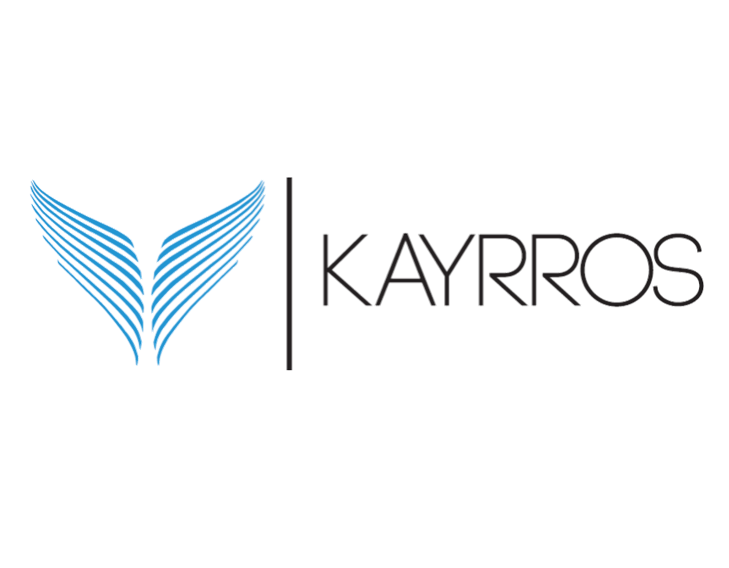
Kayyros
French-based startup founded in 2016 which is a developer of an energy analytics platform for traders, investors, operators and governments. Kayrros powers part of the Global Methane Tracker.
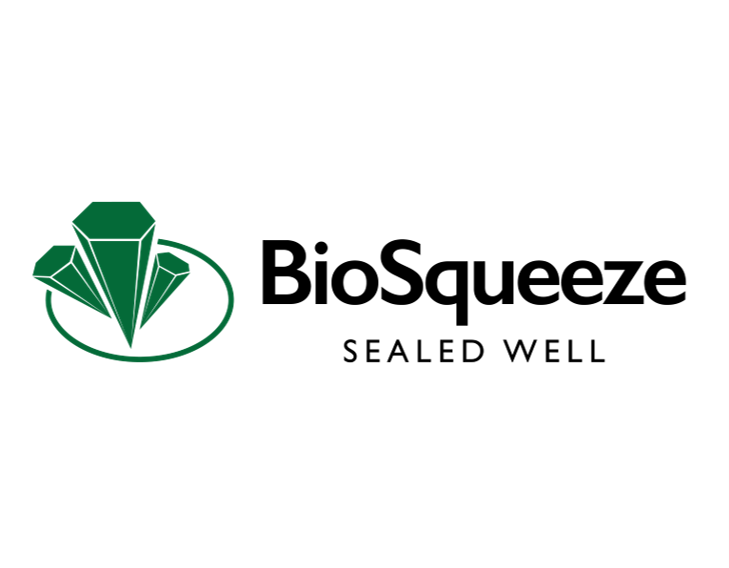
BioSqueeze
US-based startup founded in 2021 that has developed a biomineralization technology that seals miniscule leakage pathways in oil and gas wells.
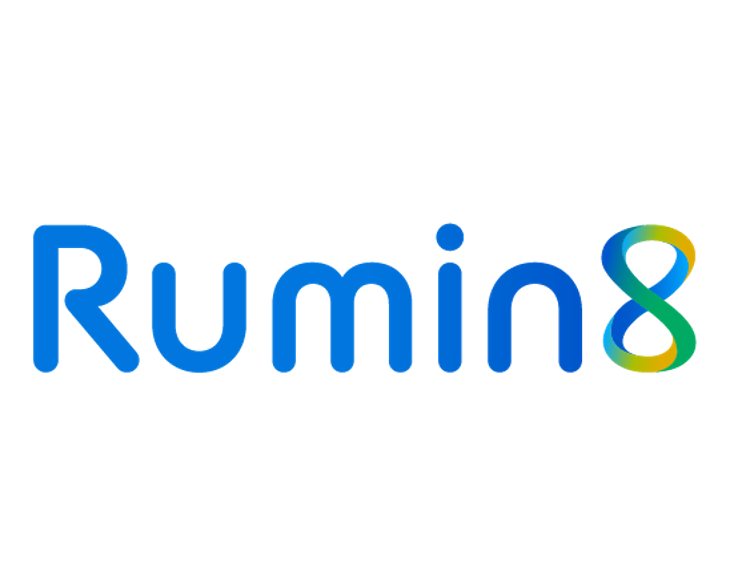
Rumin8
Australian-based startup founded in 2021 which is a manufacturer of seaweed-based nutritional supplements for livestock that inhibit methane production. The startup is backed by Bill Gates’ fund Breakthrough Energy Ventures.
Interested in a startup landscape or in an insights report?
Please fill out our contact form so that we can get back to you very quickly with our product offer.
Want to subscribe to our 123Fab?
Fill out our form to receive the latest insights into your inbox.
123Fab #98
1 topic, 2 key figures, 3 startups to draw inspiration from
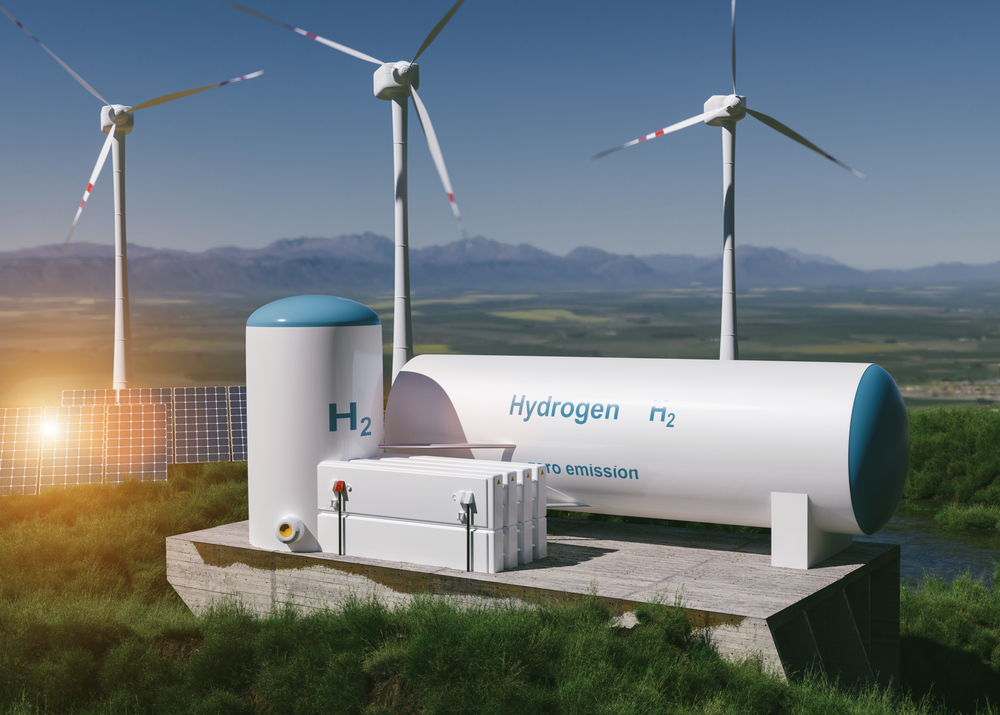
As hard-to-abate industries increasingly foster deep decarbonization strategies, green hydrogen produced from renewables via water electrolysis is expected to be at the very heart of the energy transition. However, at present, water electrolysis accounts for only about 0.03% of global hydrogen production. This is largely due to the high cost of green hydrogen (>$5/kg versus <$1.5 for grey hydrogen) due in part to the high cost of electrolyzer systems.
In this newsletter, we will examine trends in water electrolyzer innovation that reduce their cost.
Water electrolyzers are electrochemical devices used to split water molecules into hydrogen and oxygen in the presence of an electrical current. Electrolyzers are divided into four main technologies: alkaline, proton exchange membrane (PEM), anion exchange membrane (AEM) and solid oxide. Alkaline and PEM electrolyzers are the most common, produced on a commercial scale (TRL 9). AEMs are catching up in development, at TRL 6, with the development led by German startup Enapter. As for solid oxide, it is still being demonstrated with German startup Sunfire. To learn more about the technical differences, check out the IRENA report here.
Looking at the evolution of patent filings, we can detect trends in the uptake of new technologies to facilitate the implementation of large-scale green hydrogen use. Indeed, the number of water electrolysis-related patent families published worldwide has increased by 18% per year since 2005. In fact, they have surpassed the number of those related to solid, liquid and oil-based hydrogen sources. Five groups of sub-technologies stand out: (i) cell operation conditions and structure, (ii) electrocatalyst material, (iii) separators (diaphragms, membranes), (iv) stackability of electrolyzers (stacks) and (v) photoelectrolysis.
Cell operation
In an effort to improve efficiency, various electrolyzer cell operating parameters, such as higher temperature, higher pressure and zero gap cell unit design, are being explored to make them more cost-effective over a wider range of operating conditions. Danish startup Hymeth (PEM electrolyzer) has developed a high-pressure electrolyzer that operates at higher efficiency than conventional PEM technologies.
Electrocatalyst materials
Scarce materials (yttrium, titanium, iridium, platinum, zirconium) are a major barrier to the cost and scale-up of electrolyzers. Yet, the surge in patents related to non-noble metal electrocatalysts indicates that R&D is moving forward to finding new solutions to mitigate material scarcity. US startups Alchemr (AEM electrolyzers) and H2U Technologies (PEM electrolyzers) have developed electrolyzers that do not require noble metals as catalysts.
Separators (diaphragms, membranes)
Reducing the thickness of membranes increases efficiency, which in turn reduces electricity consumption. Danish startup Hystar has developed an electrolyzer that claims to reduce membrane thickness by up to 90% compared to conventional PEM technologies.
Stackability of electrolyzers (stacks)
Electrodes, bipolar plates and porous transport layers can contribute significantly to the stack cost. Improvements in these components, including scaling up their manufacturing, can lead to lower capital costs.
Photoelectrolysis
Water photoelectrolysis (water splitting using light as the energy source) is a strong, newly emerging technology. In terms of patent filings, it remains a niche technology, accounting for 6.5% of all water electrolysis patents. Yet, 37% are international patent families, which underscores the importance that applicants place on protecting their inventions outside the domestic market. A prototype of photo-assisted electrolyzer has been developed by ENGIE’s R&D laboratory CRIGEN and US startup Nanoptek.
Europe and Japan account for more than 50% of the total number of international patents in these 5 sub-technology areas. Leading players include Toshiba (JP), CEA (FR), Panasonic (JP), Siemens (DE) and Honda (JP). While Europe leads in the stackability of electrolyzers (stacks) (41% of the total patents in this area), electrocatalyst material (34%) and cell operation conditions and structure (32%), Japan ranks first in photoelectrolysis (39%) and separators (diaphragms, membranes) (36%). Chinese international patents account for only about 4% across the five technology areas but China dominates in terms of the number of pure domestic patent filings.
In short, green hydrogen technology has the potential to decarbonize numerous hard-to-abate industries. The upward trend in patent filings signals that more will soon be filed, addressing the urgent need for new solutions to lower the cost of electrolyzers, while increasing technological efficiency and production capacity. Case to be followed…
2 Key Figures
Market size of $5 billion in 2021
The global electrolyzer market size was estimated at $5.6 billion in 2021 and is expected to reach $69.1 billion by the end of 2030, with a registered CAGR of 32.21% from 2022 to 2030.
85 funded companies
Tracxn
3 startups to draw inspiration from
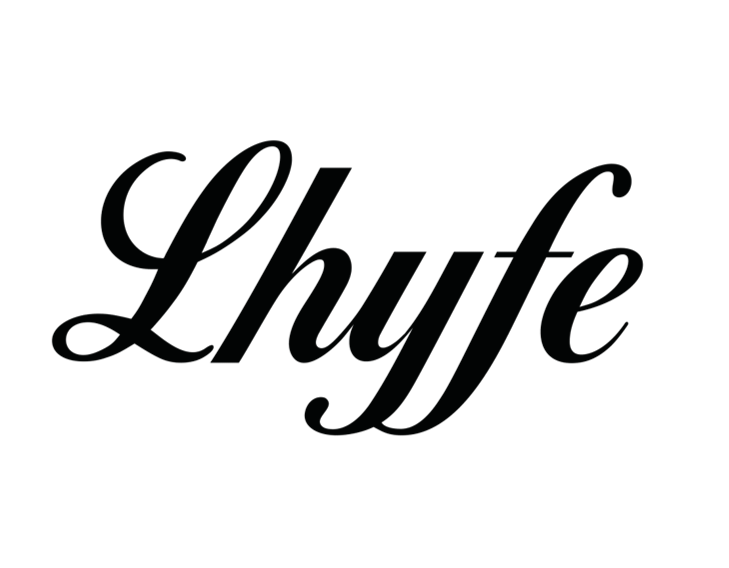
Lhyfe
French-based startup founded in 2017 which is a developer of green hydrogen plants. The first was inaugurated in 2021, connected to offshore wind turbines.
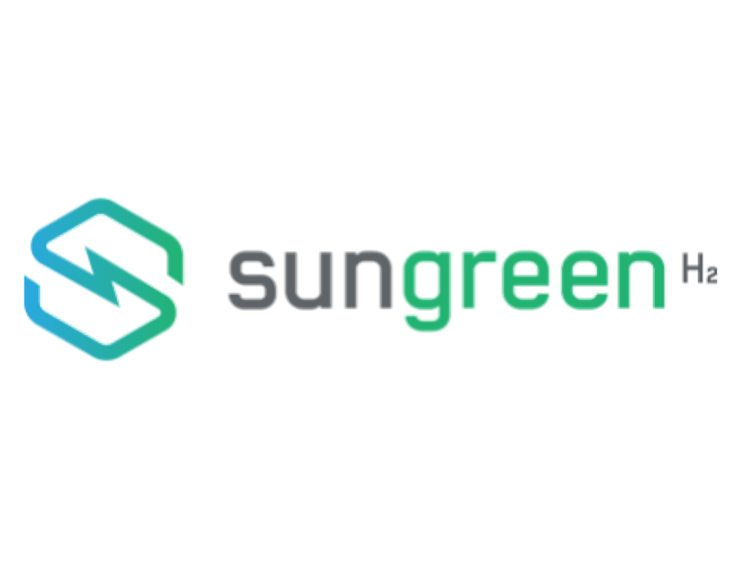
SunGreenH2
Singapore-based startup founded in 2020 which is a manufacturer of new generation components for electrolyser cells, stacks and systems. Products include PEM electrolyzers, AEM electrolyzers and solar-to-hydrogen panels.
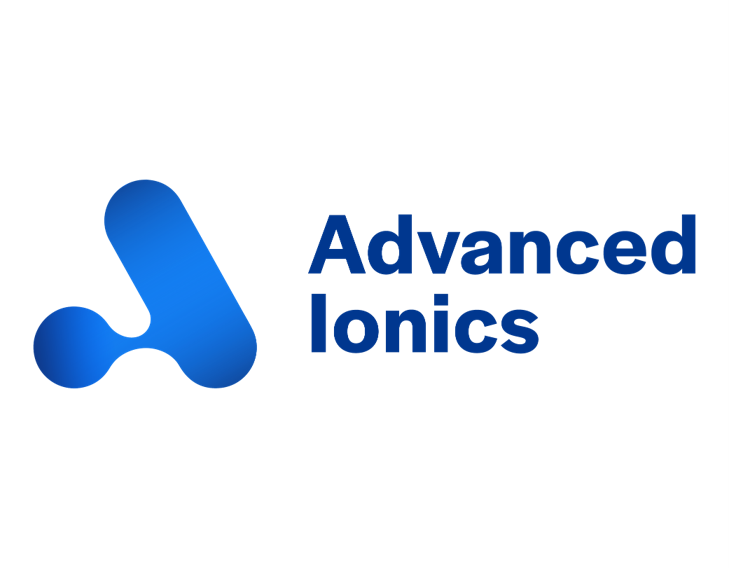
Advanced Ionics
US-based startup founded in 2016 which is a manufacturer of a new class of electrloyzers. Claims to operate at temperatures from 100°C to 650°C, in between those of alkaline, PEM and solid oxide electrolyzers.
Interested in a startup landscape or in an insights report?
Please fill out our contact form so that we can get back to you very quickly with our product offer.
Want to subscribe to our 123Fab?
Fill out our form to receive the latest insights into your inbox.
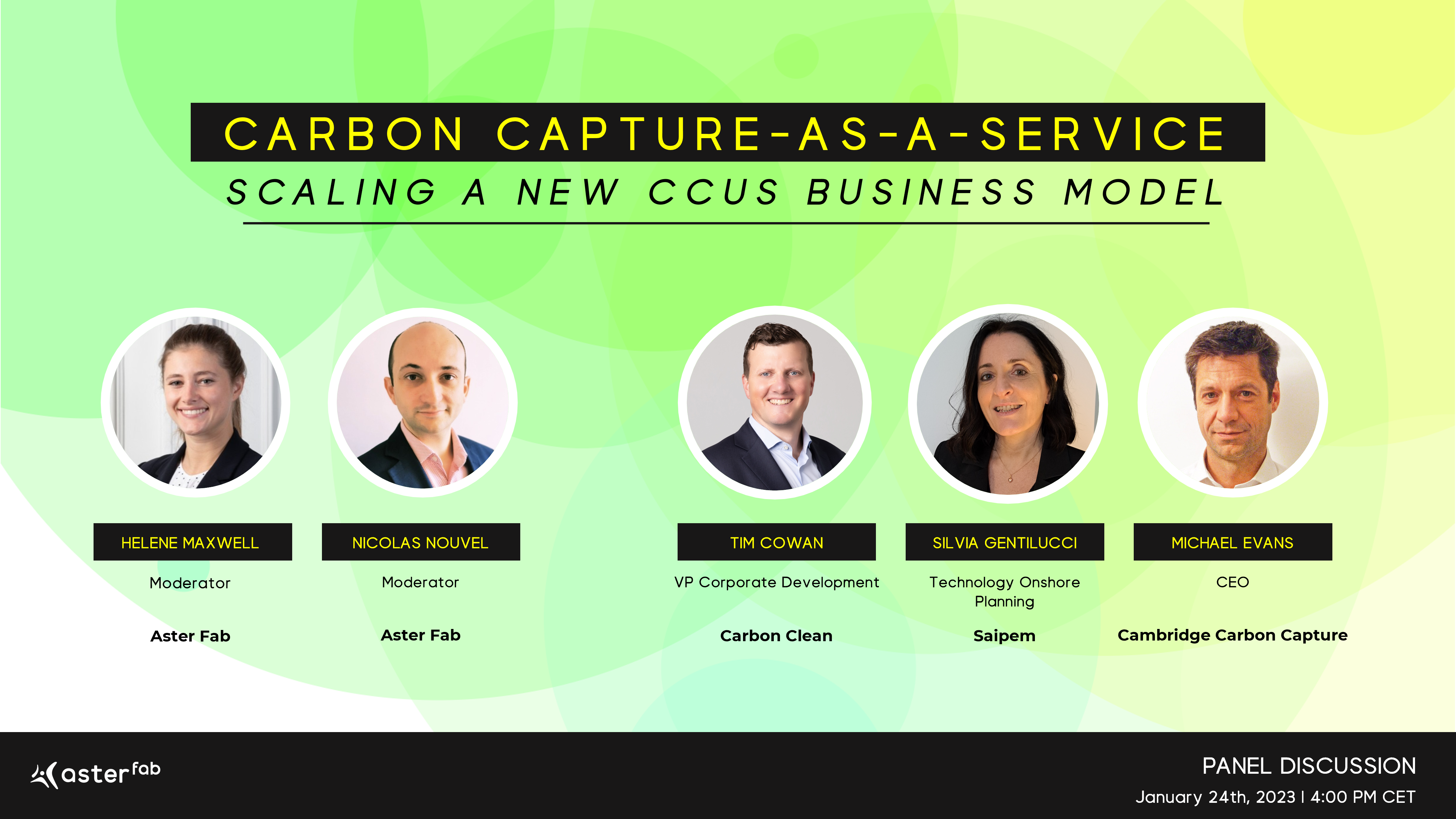
Among the numerous decarbonization solutions under development, three major carbon capture applications stand out today: industrial point source carbon capture, direct air capture (DAC) and bioenergy with carbon capture. Although industrial point source carbon capture appears to be the main focus for most decarbonization roadmaps thanks to increasingly mature and cost-effective technologies driving greater deployment across industrial sites, several challenges must be addressed before it can reach sufficient scale, including policy and regulatory support, access to funding, public acceptance and further cost improvement.
Carbon Capture-as-a-Service (CCaaS) is a business model that is gaining ground in part to circumvent the huge CAPEX hurdles encountered in these type of infrastructure projects. By opting for a one-stop shop solution that handles the entire value chain, hard-to-abate industries can pay to capture their CO2 emissions on a per-ton basis, while other specialized actors take on the risk (and potential financial reward) of managing the full value chain from capture to utilization or storage.
In January, Aster Fab moderated a panel featuring Tim Cowan (VP Corporate Development at Carbon Clean), Silvia Gentilucci (Technology Onshore Planning at SAIPEM) and Michael Evans (CEO of Cambridge Carbon Capture) to discuss the strengths and prospects of the CCaaS business model.
Takeaways from the discussion included:
CCUS adoption must increase 120-fold by 2050 for countries to meet their net-zero commitments
According to the latest Global Carbon Budget published in November 2022, if emissions are not reduced through decarbonization technologies such as Carbon Capture Utilization and Storage (CCUS), the world will have exhausted its 1.5°C carbon budget – the cumulative amount of CO2 emissions permitted over a period of time to keep within the 1.5°C threshold – in nine years. Indeed, the equation highlighted is quite simple: there are about 380Gt of CO₂-equivalent emissions left in the 1.5°C budget, and right now we use just over 40Gt of it each year.
As such, CCUS is recognized as a necessary piece of the decarbonization jigsaw, but the adoption isn’t moving fast enough. According to a McKinsey analysis, CCUS adoption must increase 120-fold by 2050 for countries to achieve their net-zero reduction goals, reaching at least 4.2 gigatons per annum (GTPA) of CO₂ captured.
Carbon Capture-as-a-Service (CCaaS): shifting capital cost to service providers, thereby allowing emitters to focus on their primary activities
In 2021, Decarb Connect conducted a benchmarking survey of industry attitudes towards CCUS that revealed that 65% of executives working in hard-to-abate industries see CCUS as ‘critical’ or ‘important’ for reaching their 2030/2050 goals. It also reveals that 41% are favorable to as CCaaS model, while 59% prefer a mix of funded and owned CCUS. In other words, no executive opted for the traditional model of owning and operating the infrastructure themselves.
Thus, the CCaaS business model appears to be a promising way to accelerate the adoption of carbon capture technology for industrial players:
- No required upfront capital expenditure
- Duty to contract with each player of the value chain is delegated
“At Carbon Clean, we use our leading technology to capture CO₂. and will work with partners to provide the other crucial elements of the value chain: compression, transportation, sequestration or utilization. Our mission is to work with industrial partners to offer an end-to-end handling of our customers’ CO₂.” Tim Cowan, VP Corporate Development at Carbon Clean.
Scaling the CCUS industry will require action by governments and investors
Tax credits, direct subsidies and price support mechanisms are beginning to encourage investment in CCUS. The US, for example, has a 45Q-tax credit that provides a fixed payment per ton of carbon dioxide sequestered or used. The IRA (Inflation Reduction Act) has increased the amount of the credit from $50 to $85 a ton for sequestered industrial or power emission, and from $50 to $180 a ton for emissions captured from the atmosphere and sequestered. In other words, they provide a direct revenue stream immediately improving the investment case for low-carbon technologies, such as CCUS. What the IRA calls tax credits, the EU calls State Aid. Yet, the panelists affirm that while the EU led the whole decarbonization movement for 30 years, the EU is now behind in terms of policy.
It is going to be very challenging for CCUS as it currently stands to make the whole thing stack up. I don’t think the carbon tax will be the viable way forward in the long-term. We need other incentives, as the US are currently doing with the IRA. Many innovative policies are starting to come out of the US and this will encourage innovative companies to set up operations there, giving the US a competitive advantage over the UK and EU in what will become a significant new industry.
There is a need to scale the whole carbon capture value chain
Another element is the uneven distribution of storage sites across Europe. Often illustrated as the ‘chicken and egg’ paradox, there is a need to scale the value chain as a whole, including storage infrastructure. Indeed, a carbon capture plant will not start operating until the captured CO₂ can be transported and then either permanently stored or used. Similarly, no large-scale carbon storage project will be financed without clear commitments regarding the origin and volume of CO2 to be stored, as it determines the financial viability of the overall project.
In Italy, there are plans to build infrastructure using depleted reservoirs in the Adriatic Sea for local storage of CO₂. Without adequate transportation and storage infrastructure, industry will not be able to adopt carbon capture technologies.
Norway’s Longship project, which is sponsored by the Norwegian government, aims to solve this problem by supporting the whole value chain from carbon capture to transportation and storage. Captured emissions will be transported by tankship and stored deep underground using Northern Light’s open-access CO₂ transport and storage infrastructure.
Garnering public support
Finally, speakers also emphasized that addressing public concerns around the safety of these technologies will be paramount. Communicating that carbon capture is safe, effective and a needed method of climate change mitigation, can help bring people on-board and ensure that projects overcome development hurdles. “I think honesty in the media about the situation would be a true incentive. If the public understood how urgent the situation is, and understood more about the technology, there would be a lot more action”. Michaels Evans, CEO of Cambridge Carbon Capture