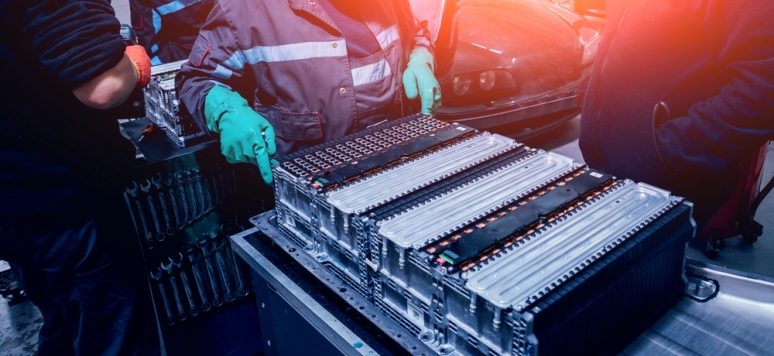
According to the International Energy Agency (IEA), the global fleet of electric vehicles (excluding two/three-wheelers) will reach 245 million vehicles in 2030 – more than 30 times the current level. But while the electric mobility revolution is underway, batteries present several challenges. EV batteries only last about 10 years and the main raw materials used in them are becoming increasingly scarce. In addition, the prices of raw metals are rising and volatile: the price of lithium in 2018 was 241% higher than in 2010, before falling in 2019. Thus, the two major consequences of this EV boom are the increase in demand for lithium, cobalt, and nickel (the key raw materials to produce battery cells) and the challenge of managing lithium-ion battery waste. Players like startup Redwood Materials, cofounded in 2017 by former Tesla CTO J.B. Straubel, are positioning themselves to address battery recycling issues. Indeed, in February 2021, Redwood Materials signed an agreement to recycle scrap and defective battery cells for Envision AESC, the Nissan car battery manufacturer in Smyrna, USA.
When an EV battery reaches the end of its first useful life, manufacturers can either dispose of it, reuse it, or recycle the valuable metals. To reuse batteries, several European automotive companies have already begun installing old EV batteries in multiple energy storage systems, ranging from small-scale residential systems to large-and grid-scale solutions. Nissan, for example, is the first carmaker to have received certification for second-life LEAF batteries to be used in stationary energy storage. Car retrofit – i.e. replacing a thermal engine with a recycled LIB or H2 battery to reduce its emissions (see our newsletter #28) – is also gaining ground. In 2019, Audi announced that it had begun testing second-life EV batteries for factory vehicles.
The other recycling solution is to separately recover the materials from the LIB to give them a second life. As resources are limited, LIB recycling seems to be essential for electric vehicles’ success. The economic benefits of battery recycling are very promising. Based on a recycling rate of 80% after 10 years of use, the profit from the battery recycling market could amount from €0.7 Bn to €1.4 Bn in 2030 (Roland Berger study).
Recycling processes generally involve a physical/mechanical separation followed by pyrolysis and/or a hydrometallurgical process.
- The pyrometallurgical process: nickel, cobalt, and copper are recovered through smelting. Despite the large energy inputs, the recovery rate of this process is low, around 30-40%.
- The hydrometallurgical process: the metal component and the recycled metal solutions are dissolved by leaching. The recovery rate of this process is higher and less-energy intensive, thus preferred by market players. For instance, the German startup Duesenfeld has built a recovery method that combines mechanical, thermodynamic, and hydrometallurgical processes. Compared to the pyrometallurgical process, their method saves 4.8 tonnes of CO2 per tonne of recycled batteries.
Both technologies are used by more and more players. Not only the traditional recycling players but also automotive companies and new startups.
Veolia has been recycling car batteries in France since 2013 and decided in September 2020 to join forces with Solvay to create a circular economy consortium to optimize the recycling of LIB for EVs and hybrids. Regarding automotive players, Audi and recycling player Umicore announced a battery recycling partnership in 2019. For this closed-loop pilot project, Umicore will recover cobalt and nickel from Audi’s e-trons (more than 90% of the cobalt and nickel in their batteries can be recycled) and process them into precursor and cathode materials to produce new batteries from recycled elements. Similarly, Singaporean startup Green Li-ion has a patented multi-cathode processor to recycle all types of Li-ion batteries into 99.9% pure cathodes. This is expected to speed up current recycling processes tenfold and increase profits fourfold.
As this recycling potential begins to be exploited by many players, legislators are supporting these trends through regulations and directives. The EU Commission, whose new Battery Directive is due to be transposed into national law by 2024, wants car and battery manufacturers to set up a comprehensive battery collection and recycling system. The stated aim is that about half the weight of lithium-ion batteries (LIB) should be recycled. In Canada, the Ontario government drafted regulations in May 2019, making the battery producer liable for the collection and management of their product to help create a circular economy. In China, the government has implemented a Battery Recycling and Traceability Management Platform in 2018, where all EV batteries are assigned a unique and traceable ID, facilitating the collection, sorting, and recycling of e-waste.
Although the regulation is being implemented, four major challenges remain:
- The inadequacy of the logistics of LIB collection channels. While this issue should gradually be resolved as recycling accelerates, the question of who will take on the role of logisticians (battery manufacturers, automotive brands, or recycling players, etc.) will remain uncertain if recycling remains centralized.
- The high upfront expenditure required to set up a large-scale LIB recycling plant. Economies of scale are likely to be seen within 5-6 years as recycling becomes more widespread and systematic.
- The requirement for a high LIB recycling efficiency rate, regardless of the LIB chemistry being recycled. The recycling rate is already around 80% and is expected to increase in the coming years, as some startups are developing prototypes capable of recycling more than 90% of the LIB.
- The recycling steps vary according to the different types of batteries (lead-acid, nickel-cadmium, zinc-air, alkaline, …). Thus, recycling facilities need to be adapted to several processes. Even among LIB batteries, the most used for EVs, the process differs according to their composition and recovery rate.
To conclude, lithium-ion batteries are likely to remain the major battery type for EVs in the coming decades (due to their high energy intensity relative to their weight, compared to other batteries such as lead-acid, nickel-cadmium, or zinc-air). There is a need to close the loop to meet the demand for their scarce and expensive raw materials.
2 Key Figures
51 battery recycling startups
registered by Traxcn
Market size expected to reach $12.2Bn by 2025
The size of the battery recycling market is expected to reach $12.2 Bn by 2025, compared to a market of $1.5 Bn in 2019.
3 startups to draw inspiration from
This week, we identified three startups that we can draw inspiration from: Li-Cycle, Green Li-ion and Lohum.
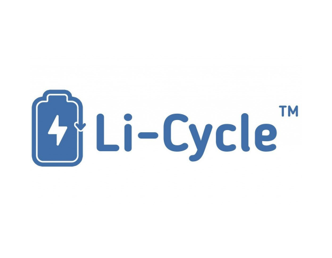
Li-Cycle
Li-Cycle is a Canadian startup that uses a combination of mechanical size reduction and hydro-metallurgical resource recovery techniques to recycle lithium-ion batteries. Their recycling technique does not generate any hazardous product which minimizes transportation liability and lowers costs.
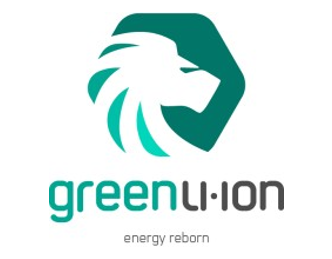
Green Li-ion
It is a Singapore-based start-up which recycles the metals of LIBs (cobalt, nickel, manganese and other expensive metals), recover them, and make them ready to be reused in new batteries. Their patented technology is cleaner, faster, and four times more profitable than current LIB recycling. It is also the only technology to fully rejuvenate the battery cathode
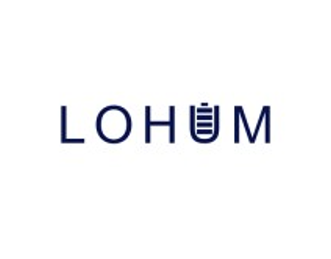
Lohum
Lohum is an Indian start-up manufacturing of Li-ion battery packs and recycling battery components. It provides full lifecycle solutions for manufacturers involved with low-power mobility and storage applications such as electric two-wheelers, rickshaws and inverters. It offers secondary life cycle solutions such as battery recycling to large manufacturers and companies using Li-ion batteries.
123Fab #36
1 topic, 2 key figures, 3 startups to draw inspiration from
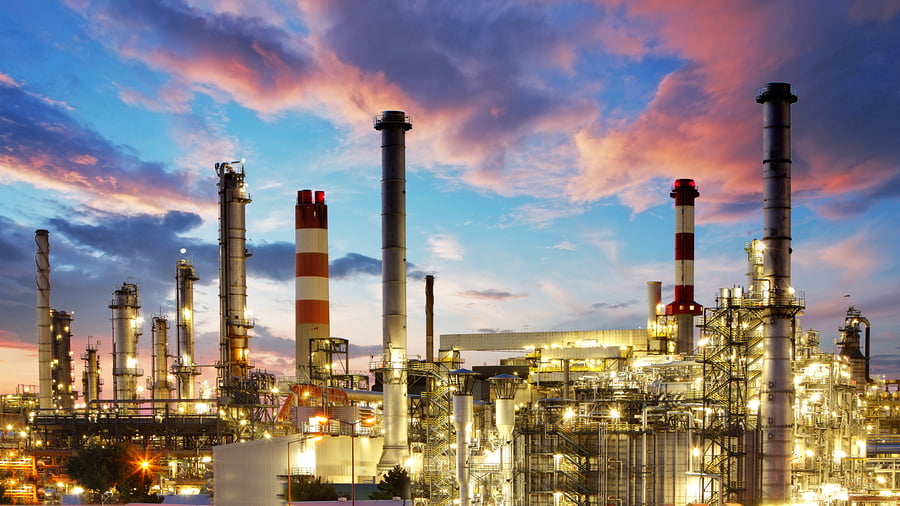
On the one hand, heating and cooling applications are among the largest energy consumers (about half of the total energy consumption). On the other hand, some of the renewable electricity produced is lost due to non-immediate use, and this will continue to accelerate as renewable energy facilities are being developed faster than batteries and storage solutions. The reconciliation of these two issues, converting electrical energy into heat (or cold), is called Power-to-Heat (PtH). Thermal energy is produced by heat pump technologies or electric boilers. In the context of growing environmental awareness, industrials are increasingly looking for technologies that will enable them to shift from fossil-based industrial heating to electrically-based, power-to-heat processes. These industrial heating applications account for almost 20% of global energy consumption.
These renewable power-to-heat technologies help industries to reduce their CO2 emissions and offer higher flexibility in the power system when equipped with smart load management. Industrial application technologies are already mature and commercially available. However, they have yet to be integrated into hybrid heating systems (e.g. with natural gas).
How does it work? The first step is the delivery of electricity from renewable sources to power stations (which may be large centralized heating production stations or decentralized entities). Infrastructures can be equipped with thermal storage systems, such as those of Form Energy, to enable consumers to use the stored heat and thus reduce the demand on the power grid during peak electricity demand periods. Afterwards, there are two technologies to convert electric power into heat: electric boilers and heat pumps. Electric boilers use electricity to heat water, which is then circulated through pipes to provide space heating or stored in hot water tanks for later use. Heat pumps, on the other hand, transfer heat from the surrounding heat sources to buildings and infrastructures. They can fulfill both heating and cooling requirements — typically using between 66% and 80% of the energy contained in the ambient air, water, or ground, and between 20% and 33% electricity to drive the process.
Where is it used? These technologies are applied in different industries, for several uses. The first example is the food & beverage industry, where heat plants are used in Japan for brewing sake and beer. The Suntory production plants, for instance, use a cogeneration system (combined heat and power) that recovers the heat generated from in-house generation and uses it as a heat source for brewing beer and extracting coffee and tea, increasing energy efficiency to 70-80% and reducing CO2 emissions by 20-30%. Another example is the container washing plant in Spain that uses solar thermal heat. 22% of their hot water (80°C) demand is covered by a solar thermal system based on flat plate collectors, and the remainder is covered by a conventional boiler using natural gas. District heating is also a common application of power-to-heat. In Hamburg, Vattenfall operates an electric boiler that uses excess wind generation, thus avoiding wind power curtailment, to generate district heat in Berlin. The units use electricity from renewable energy sources to heat water, which transmit heat to residences and commercial buildings.
More generally, power-to-heat innovations contribute to the transformation of the power sector in 5 ways:
- Reduction of renewable energy curtailment: the excess of energy is used to address heating needs.
- Increased flexibility through load-shifting: heat pumps can offer demand-side flexibility by switching their electricity consumption from high-demand time intervals to low-demand time intervals to convert electric power into stored heat or cold.
- Large-scale energy storage: the surplus heat (resp. cold) produced with renewable energy in summer (resp. winter) can be stored in thermal reservoirs (mainly aquifers), which then can be used to meet the winter (resp. summer) heating demand, thereby reducing the need for non-renewable heat sources during peak times. The most common solution is the use of Phase-Change Materials (PCM), which are efficient against energy loss and leakage and are substances that release or absorb enough energy to maintain a regulated temperature. A great example of such a process is the Canadian project Drake Landing, which uses solar thermal energy and seasonal underground thermal energy storage for a district heating scheme. It supplies a residential community of 52 households that have seen their greenhouse gas emissions cut down by more than 5.5 times per year.
- Grid services provided by aggregators: new “smart” storage heating solutions are designed to take advantage of variations in electricity prices throughout the day and can be remotely controlled by aggregators to both optimize heating costs for consumers and provide grid balancing services to the national grid.
- Increased self-consumption through renewable local generation: consumers with solar rooftop systems can use the locally generated electricity to power heat pumps.
Ultimately, having understood how power-to-heat systems work and what their benefits are, it can be useful to bear in mind the drivers behind their adoption and the regulations that are put in place. Incentives to decarbonize the heating sector are leading to the deployment of heat pumps at a steady pace. On average, the operating costs of using electricity to generate heat are comparable to those of using fossil fuel-based sources. High-performance heat pumps can generate more than 4–5 kWh of useful heat for every 1 kWh of electricity consumed. Furthermore, regulations are being implemented in pioneer countries, like in Germany, where the Renewable Energy Heating Act bans the use of oil burners to heat new buildings and requires all new buildings to use energy generated from renewable energy sources for space and water heating. Similarly, in 2017, Norway’s Ministry of Climate and Environment passed a law banning the use of oils and paraffin from 2020 in heating applications.
To conclude, now that power-to-heat technologies are mature and up-and-running, more incentives should be brought forwards to increase the use of renewable energy in heating and cooling. Domestic and industrial consumers will need to make upfront investments to shift to renewable energy for heating and cooling applications, and schemes that reduce the economic burden on consumers will encourage faster adoption of renewable energy in heating and cooling. However, these schemes need to be tailored to the needs of different consumer segments, types of buildings (residential vs. industrial), and types of heating system (centralized vs. decentralized), as well as to other external factors, such as the climate zone.
2 Key Figures
177 power-to-heat startups
registered by PitchBook, including 100 “thermal energy storage” startups
Market size expected to reach $369M by 2025
The market size of thermal energy storage is expected to reach $369M by 2025, at a CAGR of over 14.4% from 2020.
3 startups to draw inspiration from
This week, we identified three startups that we can draw inspiration from: Malta Inc, Enerstorage, and Heaten
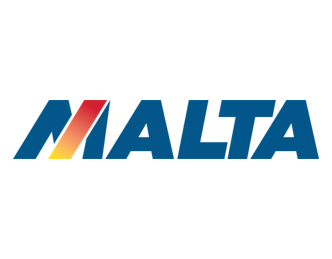
Malta Inc
The US-based startup Malta Inc builds an electro-thermal energy storage system that converts electricity to thermal energy for storage. It later converts the thermal energy back into electrical energy whenever required
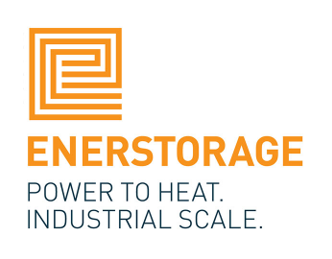
Enerstorage
The German startup Enerstorage sells power-to-heat plants for industries that require a lot of heat. The PtH systems provide an important link between the heat supply and the power grid, regulate the power grids, and thus lead to a successful energy transition.
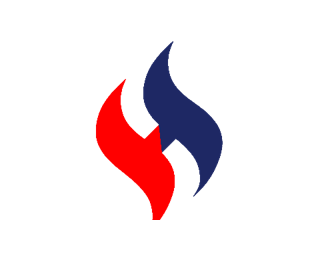
Heaten
Heaten is an industrial startup that provides heat-to-power and power-to-heat machines. Their very high-temperature heat pumps are based on an innovative piston machine technology, which provides an output temperature up to 165°C, which covers 30% of the energy demand of all industrial heating processes.
123Fab #35
1 topic, 2 key figures, 3 startups to draw inspiration from
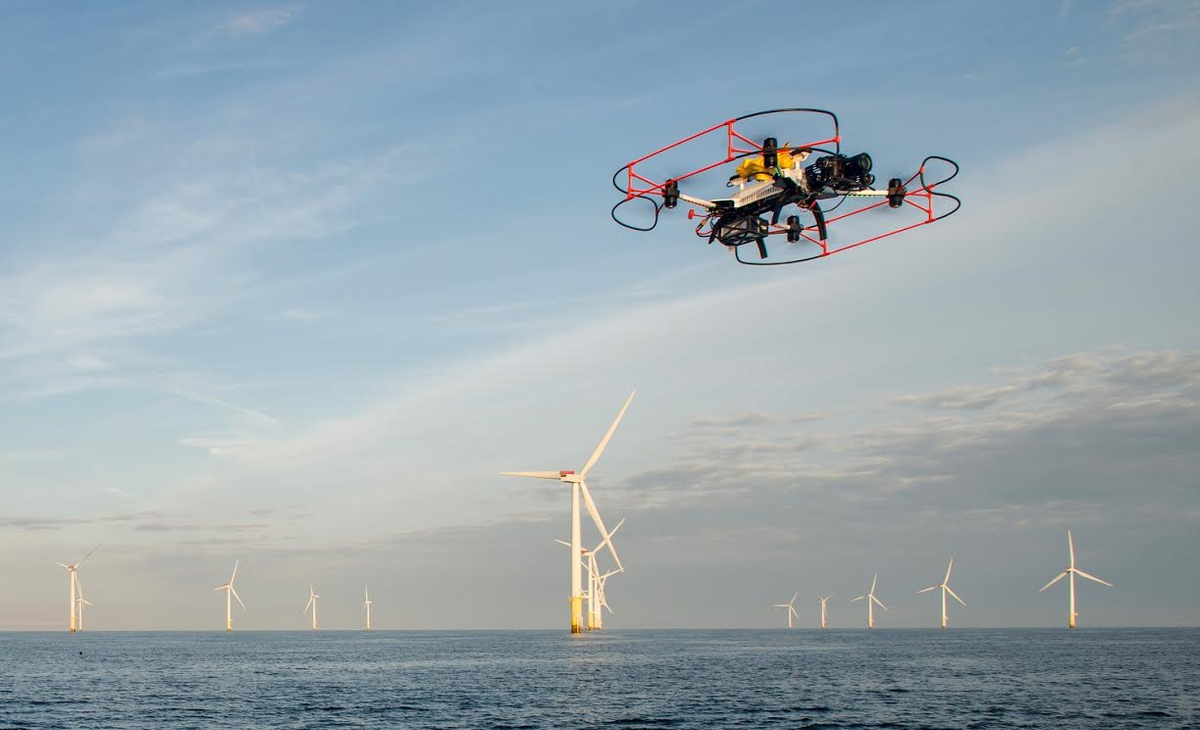
In February, Estonian start-up Hepta Airborne raised €2 million to take its drone powerline inspection solution to the next level. Using LiDAR, thermal sensors and cameras, Hepta Airborne helps automatically detect powerline and power infrastructure defects. This fundraising embodies the current surge in the use of drones for difficult tasks in the industry. Indeed, the overall commercial drone market is projected to reach $43 billion by 2024, up from $587 million in 2016. The volume of VC investments has increased by 21% over the past 4 years, reaching $185 million in 2020. In the coming years, the market is expected to become more concentrated, with the leaders winning out over weaker players, as did the fast-growing start-up Airobotics, which raised a total of $123M in funding over 5 years.
Drones are used in a variety of industries, but above all in 4D situations: dirt, dull, distant, and dangerous. The main industries that use them are the energy sector (both oil & gas and renewables), precision agriculture, construction, and mining. Although regulations are often a hindrance, there are gradually being adapted to each industry and use case, in order to enable the effective use of drones.
The energy sector can greatly benefit from drone inspections, which not only help to reduce costs, but also to prevent disasters and save lives. Indeed, they allow distant and dangerous inspections to be carried out, eliminating the need for climbing wind turbines or reaching offshore oil platforms. Although standards for drone operations are yet under development, they could help expand the use of unmanned aircraft services in the energy industry and boost innovation. The main challenge, however, lies in flights beyond the visual line of sight (BVLOS) – to carry out pipeline and powerline inspections over long distances, for instance – as the detect-and-avoid technology is not sufficiently advanced. Improvements in advanced EO/IR [electro optical/infrared] sensors, acoustic sensors, machine learning, ground-based radar, and other technologies could change the game. Avitas Sytems, a General Electric venture, has developed a digital platform and drone inspection capabilities for pipelines, for instance. Wind turbine inspection can be monitored by drones, such as those of the start-up Aerialtronics.
Drone activity in agriculture continues to increase, and the aerial imagery generated can provide unique insights by scouting crops, reporting crop damage, or determining tile locations. Drone use is mainly justified by a more accurate collection of crop data and the avoidance of dull stains. Over the past ten years, the Federal Aviation Administration (FAA) has continued to review the requirements for the operation of small unmanned aerial systems to create a reasonable legal pathway for use in agriculture. This involves obtaining a remote pilot certificate, registering the drone with the FAA, but also weighing less than 55 pounds, maintaining a maximum altitude of 400 feet, and remaining within the visual line of sight of the remote pilot or visual observer in command. Although these regulations seem restrictive, they enable farmers to use them as part of their needs. The Swiss start-up Gamaya uses HSI (Hyperspectral Imaging) technology deployed using small unmanned aircraft systems for remote sensing and high-resolution imagery. It can be used to diagnose crop diseases, the proliferation of invasive species, and environmental stresses.
As for drones in the construction industry, they are mainly used for surveying and inspection purposes. They perform dull, dangerous, distant, and time-consuming tasks. Drones are equipped with downward-facing sensors, such as RGB, multispectral, thermal, or LIDAR, and capture a large amount of aerial data in a short time. According to a PwC study, the use of drones throughout a construction project provides an unparalleled record of all activities; cuts planning and survey costs; increases efficiency and accuracy and eliminates disputes over the status of a project at a given point in time. Drafted regulations in the construction industry frame the use of drones, without preventing it. Drones can only fly during daylight, must be close enough to the operators to be seen by the naked eye, and cannot exceed a certain altitude and speed. The Swiss start-up Wingtra, which has raised a total of $19M, provides mapping drones for construction sites.
Finally, drones in the mining industry help solve challenges such as better blast optimization, improved safety, faster surveying, and the construction of the most comprehensive and continuous project datasets. On mining sites, drones are used to cover distant areas where foot traffic is not allowed. Their aerial photography and remote sensing allow mining companies to capture all that information without putting someone at risk.
All these examples highlight the significant potential of drones in the industry. Apart from regulation issues, the main factors limiting the massive adoption of drones are technical issues (battery autonomy, drone fleet management, data transfer, etc.) and practical issues (lack of certified pilots, hence the creation of marketplaces for drone rides).
To conclude, the potential of the drone market is high and has not yet reached maturity, and private investors are betting on it. Harmonization of regulations is underway – for recreational drones, the EU announced a continent-wide standardization on January 1st; and technology innovations (battery life, collision avoidance, autopilot, data processing, control & communication systems) should follow to enable democratized use in the industry.
2 Key Figures
1,009 drone startups
registered by Tracxn
Market size expected to reach $43bn by 2024
The market size of commercial drones is expected to reach $43bn by 2024, a CAGR of over 20% from 2018
3 startups to draw inspiration from
This week, we identified three startups that we can draw inspiration from: Percepto, Asylon, and SkySpecs.
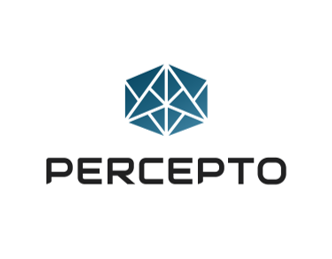
Percepto
Percepto is a developer of autonomous drone technology for inspection and surveillance. The company has developed solution to holistically inspect and monitor industrial sites, harnessing remote robotics to autonomously collect, aggregate, and analyze visual data. Percepto operates in mining, oil & gas, industrial sites, and solar energy production sites.
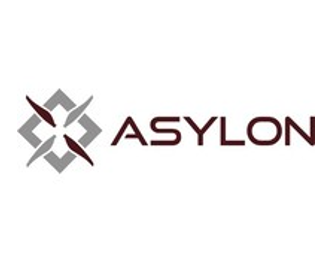
Asylon
Asylon manufactures and distributes a range of field deployable infrastructure to its clients. Among others, the company manufactures DroneHome, a field-deployable battery swap station. It offers data linking, coverage, mesh networking, and mixed fleet support. Asylon has chosen a robot-as-a-service model, where they provide an end-to-end solution for an annual subscription.
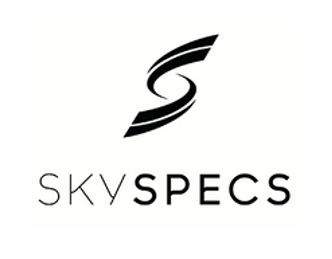
SkySpecs
SkySpecs is a provider of autonomous drone inspections for onshore and offshore wind turbines. The safety software services include the development of an automated drone inspection feature for applications in wind turbines, utility and other infrastructure operations and maintenance activities and provides an analytics platform that supports workflows at every level of the value chain.
123Fab #34
1 topic, 2 key figures, 3 startups to draw inspiration from
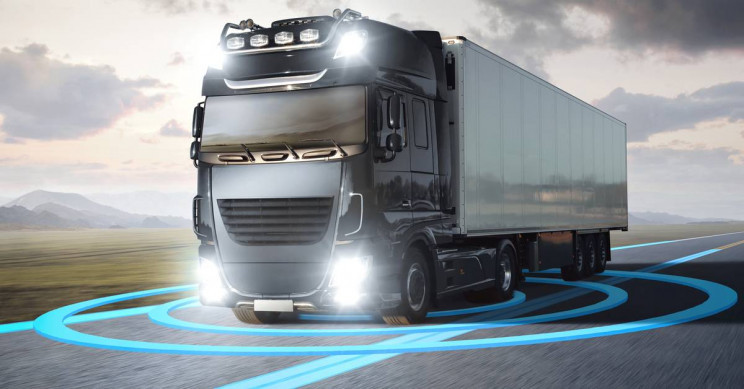
Breakthroughs in the field of autonomous vehicles, including for freight, have leaped forward in recent years. In October 2020, Swedish startup Einride unveiled a new driverless vehicle for autonomous freight, which the company hopes to see on the road this year. Last year, Plus.AI completed its first coast-to-coast commercial journey across the US with an autonomous truck, using simultaneous localization and mapping (SLAM) technologies. Indeed, a combination of factors has been fueling this development, including the increasing road freight (with 11,000 bn ton-km today, it is expected to double over the next 20 years) and the shortage of drivers (a lack of 175,000 drivers by 2024, according to the American Trucking Association).
In this respect, the European Commission launched in December 2020 a 29-partner consortium – All-Weather Autonomous Real Logistics Operations and Demonstrations (AWARD) – to shake up transport for the freight industry, and in particular, “operations in difficult weather conditions”. Led by EasyMile, this 3-year, €20 million project brings together truck manufacturers, equipment manufacturers, end-users and logistics operators.
While institutions are beginning to support autonomous freight, innovation and new technologies are the playground for startups and established automotive players. Innovations can be split into four main areas: sensors such as cameras or lidars (which provide data to a trained computer), computing hardware (responsible for autonomous driving calculations and decision making), storage infrastructure (to store data, on the edge and in the cloud, for future analysis and algorithm improvement) and autonomy software (which ultimately takes all the decision). If the range of technologies is similar to that of autonomous cars, long-distance trucks are better suited to test automated driving because of their extensive use of highways – where unexpected situations are less likely to occur than on urban roads – and the repetitiveness of their itineraries.
Startups at the wheel, which work on more or less autonomous trucks, include:
- US start-up TuSimple, a developer of autonomous driving technologies applied to trucks. It already has partnerships with two OEMs (Navistar and VW’s Traton truck OEM) and plans to reach Level 4 autonomy (trucks able to operate without a human driver under limited conditions that may include the time of day, weather, or pre-mapped routes).
- Otto, which was founded by former Google, Tesla, and Apple employees, is developing software to be installed on existing trucks to make them fully autonomous.
- Embark Trucks has already commercialized fully automated semi-trucks and trucks (used by Amazon for some loads).
Automotive and logistics players are also following the trend:
- Daimler Trucks signed a partnership with Waymo last October to deploy autonomous SAE L4 technology. They will combine Waymo’s cutting-edge automated driver technology with a unique version of Daimler’s Freightliner Cascadia, which will be commercialized in 2025.
- In 2016, a fleet of semi-autonomous Scania trucks (a Swedish manufacturer specializing in heavy vehicles) completed a journey from Sweden to the Netherlands using a technique called platooning, in which one driver pilots the leading vehicle while the rest follow along automatically (such as the one developed by Peleton Technology)
- Walmart announced last December that it would test driverless autonomous delivery trucks with startup Gatik from 2021. The trucks are equipped with sensors and autonomous driving software. To limit the risks, they have decided to start driving on the same and registered routes (mainly between warehouses and supermarkets).
The recent and on-going acceleration in autonomous freight vehicles is due to the many advantages they provide. 45% reduction in exploitation costs (McKinsey), better utilization (they operate 24/7 without driver rest periods), and a higher payload of trucks (as there is no driver cabin anymore). Moreover, it improves drivers’ conditions (avoiding night drives, more rest thanks to driving assistance). Finally, autonomous vehicles contribute to higher road safety (security systems should avoid 90% of crashes caused by human error) and a reduction of carbon footprint thanks to methods like platooning or IoT to optimize fuel consumption.
Nonetheless, several challenges or competitive alternatives remain. The impact on jobs is difficult to assess, due to the higher demand and need for monitoring of self-driving trucks. Autonomous fleets face autonomous trains which have higher productivity. One could see an opportunity for railway companies to integrate automated trucks. They could increase speed and throughput as well as secure a critical role in the overall ecosystem. Finally, as with autonomous cars, regulations need to be harmonized between countries, and the issue of liability is essential for further deployment.
To conclude, the market is growing fast and innovations are multiplying, which will likely lead to a technology transfer to other autonomous devices (cars, machines, robots, etc.). Institutions and key players, as Uber does, still need to work on driverless vehicle and regulation issues.
2 Key Figures
30 self-driving truck technology-provider startups
registered by Tracxn
Market size expected to reach $2.01bn by 2027
The market size of autonomous trucks is expected to reach $2.01bn by 2027, a CAGR of 12.6% from 2019
3 startups to draw inspiration from
This week, we identified three startups that we can draw inspiration from: Plus.ai, Kodiak Robotics, and Boxbot.
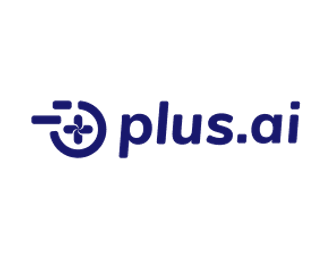
Plus.ai
Plus.ai develops self-driving technology for trucks. It offers solutions like the 360-degree perception that uses radars, LiDARs, and cameras for sensing. Their localization & mapping algorithms accurately track the location of trucks and detect and analyze road structures, and predict the behavior of other on-road vehicles.
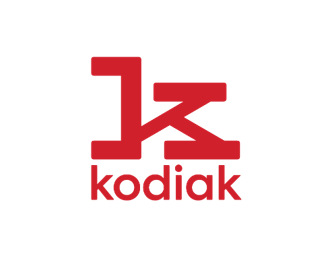
Kodiak
Kodiak Robotics is a developer of self-driving trucks. It develops autonomous trucks with a full-stack solution with simulation enhanced system to optimize the risk. Their fleet of self-driving trucks ahave been tested in California and are currently operating in Texas.
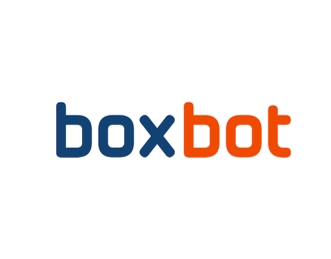
Boxbot
Boxbot is a developer of automation technology designed to address the last-mile issues in logistics, enabling businesses and individuals to optimize the experiences of suppliers and consumers.
123Fab #33
1 topic, 2 key figures, 3 startups to draw inspiration from
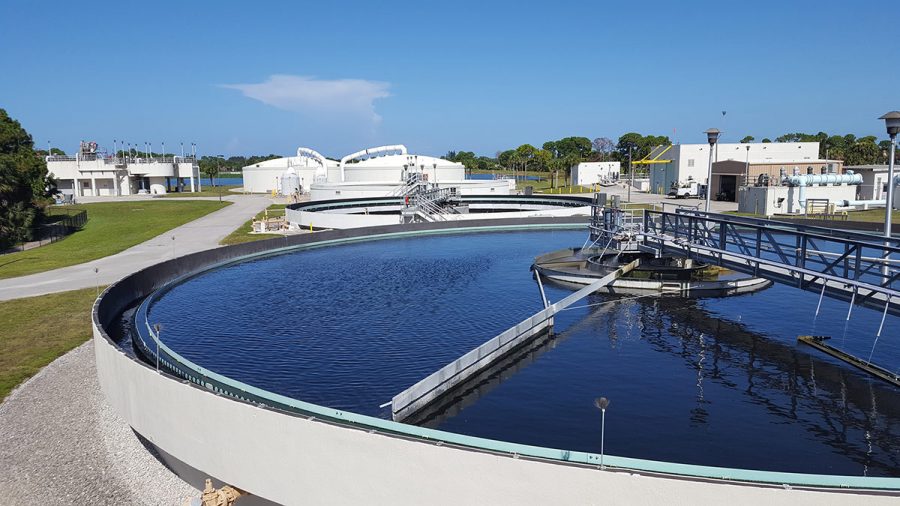
In January 2021, a research team from the Institut National de la Recherche Scientifique (INRS) developed an electrolytic wastewater process that degrades microplastics at the source, instead of a filtration process. Indeed, microplastics can be very present in wastewater, particularly in industrial waster. Industrial wastewater treatment describes the processes for treating wastewater produced by industries as an undesirable by-product.
Given the current pressure on water resources, water contamination issues and the water needs of industries (22% of global use, and 40% in Europe), industrial wastewater treatment is becoming imperative. All the more so as many industries are concerned by contaminated wastewater, be it agriculture (fertilizers), the construction industry (solvents, paints, grease), the food processing industry (organic particulates, animal waste) or oil & gas sectors (grease, oil waste). Treating industrial wastewater has two main benefits, which are reducing industries’ environmental footprint (by reducing waste and harmful contaminants released into the environment) and contributing to the circular economy (by reusing the wastewater to produce energy or fertilizer), thus turning waste into an additional source of revenue. Thereby the market is becoming more competitive and fragmented. However, the industry remains dominated by large companies, relying on their brand and patented product technology. Among the main players are the French companies Suez – which invests €74 million each year in more R&D programs for wastewater treatment solutions — and Veolia, but also international corporations like Ecolab (US) or Thermax Group (India).
The treatment of industrial wastewater requires special methods and technologies that can be divided into three categories:
- Physical treatment methods used to remove solid waste. The main techniques are sedimentation (suspending the insoluble particles from the water and separating them once they have settled to the bottom), aeration, and filtration.
- Chemical treatment methods involve the use of chemicals in the water. Chlorine and ozone are oxidizing chemicals commonly used to kill bacteria and prevent them from reproducing in the water.
- Biological treatment methods use a variety of processes to break down the organic matter present in wastewater. They include aerobic processes (a bacteria decomposes the organic matter and converts it into CO2 that can be used by plants), anaerobic processes (fermentation of the waste at a specific temperature), and composting (wastewater is treated by mixing it with sawdust or other carbon sources).
These three techniques to treat industrial wastewater are used at different stages of the purification process. During the screening phase, physical processes like sedimentation and filtration are used to remove solid waste from the wastewater. For instance, biofiltration is used to ensure that any additional sediment is removed from the wastewater. This is the core of Veolia’s patented technology, Biostyr. The wastewater is then clarified, using physical or biological methods, to remove suspended and floating matters. The refinery industry, for instance, uses a clarifier with a surface skimmer for oil and a bottom rake for solid – also called API separator. When the difference in density is not sufficient to separate oil-wetted solids, air floatation can be used. The company Akvola sells a solution based on a bubble generator and a flotation-filtration process for cleaning hard-to-treat industrial wastewater. The air bubbles attach to contaminants in the waste, decrease their density, and facilitate their separation. Afterward, wastewater is aerated (introduction of air into the water) to allow aerobic biodegradation of the polluting components. Finally, industrial wastewater is disinfected by chemical or biological processes. When it comes to final sewage sludge, it is treated before being sent back to the environment or reused. Sewage sludge is first thickened, then digested and dewatered. During digestion, the sludge produces CO2 and methane, often collected and used to generate power, as is done by AquaGreen. They provide products that focus on converting wastewater sludge into thermal energy, capable of supporting sludge facilities and district heating.
To conclude, innovation and technologies are being developed to treat increasing amounts of industrial wastewater. The environmental footprint (sewage sludge) and energy consumption (2-3% of global energy for water purification) are challenges that need to be addressed in order to reach maturity.
2 Key Figures
98 Industrial wastewater treatment startups
registered by Tracxn
Market size expected to reach $15bn by 2024
The market size of industrial wastewater treatment is expected to reach $15bn by 2025, a CAGR of 5.8% from 2019.
3 startups to draw inspiration from
This week, we identified three startups that we can draw inspiration from: ProSep, Akvola, and EcoWorth Tech.
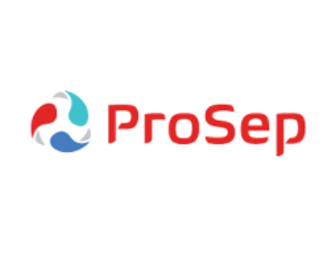
Prosep
Prosep offers several solutions to Oil & Gas businesses. They have a variety of tertiary wastewater treatment technologies, aimed at achieving and maintaining low levels of harmful contaminants in the water streams produced by discharges. Their wastewater treatment solution can be retrofitted into the existing treatment line.
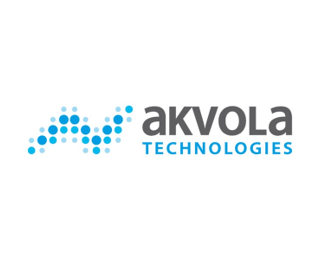
Akvola
Akvola sells environmentally-friendly solutions based on the proprietary MicroGas Fine Bubble Generator and akvoFloat – a flotation-filtration process– to clean hard-to-treat industrial wastewater containing high concentrations of oil (free and emulsified) and suspended solids. It can remove up to 99% of oil & grease and suspended solids
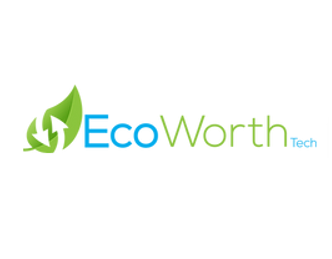
EcoWorth Tech
EcoWorth Tech is a Singaporean startup providing waste-to-worth solutions. They are specialised in transforming waste materials of industrial processes, oil & gas industry and food processing, into reusable products. They notably rely on their “Carbon Fibre Aerogel” technology to treat wastewater.
123Fab #32
1 topic, 2 key figures, 3 startups to draw inspiration from
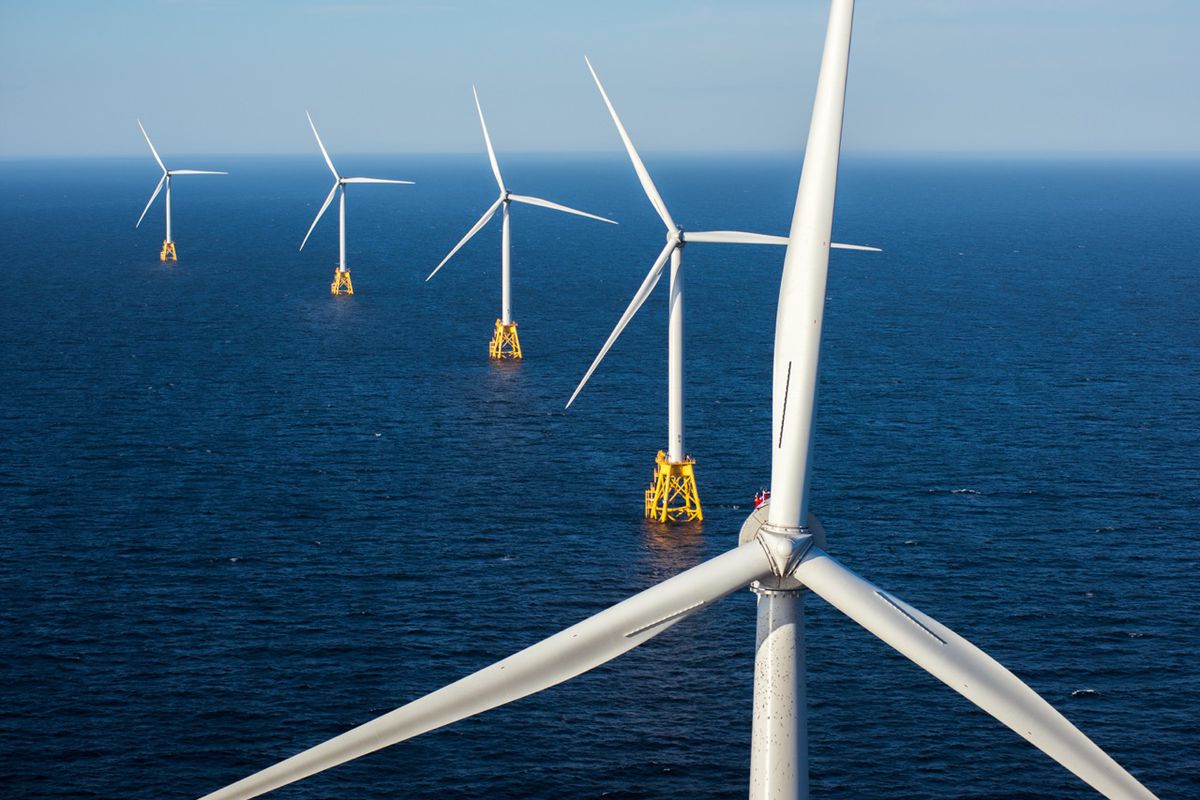
While offshore wind stagnated under Trump, President-elect Joe Biden’s policies could create a clean energy surge. Indeed, Joe Biden’s climate plan proposes building thousands of offshore wind turbines to put the US on a path to carbon-free electricity by 2035. On the other side of the Atlantic, the EU Strategy on Offshore Renewable Energy, published in November 2020, also acknowledges that harnessing the energy potential of European waters is crucial to meeting climate targets, and estimates that at least 60GW of offshore wind capacity will need to be installed by 2030 (compared to 18.5MW installed today).
Offshore wind farms are able to generate more electricity at a steadier rate than onshore wind farms thanks to higher and more consistent wind speeds. If the wind blows stronger offshore, the total cost of energy (installation, material costs, etc.) is higher, and the operations (grid connection, etc.) are more complex than onshore wind turbines. The growing attractiveness of the offshore wind market can be explained by all the technological improvements that have made it possible to increase turbine capacity and reduce costs.
The technology developments in the offshore wind sector span across the entire value chain:
- Wind measurement: If met masts (steel towers with measuring equipment on top) are the standard for onshore wind turbines, LiDAR (Light Detection And Ranging) solutions are best suited for measuring wind speed on offshore installations. They can remotely measure distances to a target using lasers, are easier to install and maintain, cheaper and more reliable, and safer for workers. According to the Danish company Windar Photonics, they can even increase energy production by 1 to 4%. This manufacturer of LiDAR solutions sells, among others, WindEYE, a two-beam radar (80m) mounted on a wind turbine nacelle, that corrects any misalignment.
- Turbine: Several types of turbine innovations are underway. First of all, efforts are being made to increase the speed of the blade tips (there is no noise problem as there is with onshore wind) from 70-80 m/s to 90-100 m/s, to generate more power. Further efforts are being made on rotor design, with 2 blades instead of 3, which allows manufacturers to reduce costs and downwind designs are suitable in regions with typhoons. These turbines can be found in the port of Eemshaven, in the north of the Netherlands.
- Foundation and support structure: New concepts to reduce installation costs and improve safety are being developed. A 3-legged jacket design, instead of 4, facilitates installation while reducing material costs. When it comes to the foundations, screw piles are being replaced by suction bucket technologies. Water is pumped out of upside-down buckets, thereby creating enough differential pressure to hold the foundation to the seabed. This technique was first used in 2018 for the European Offshore Wind Deployment Centre (known as Aberdeen Offshore Wind Farm). Self-installing gravity foundations are also being tested as they limit costs by reducing the use of heavy equipment vessels. The foundations are produced on land, towed to the wind turbines and then immersed with water and sand to rest firmly on the hard seabed.
- Operations and maintenance: Automated inspection by drones, instead of being performed manually, is expanding. For instance, the startup Skyspecs offers an aerial blade monitoring drone solution that can complete a turbine inspection in less than 15 minutes. On top of increasing safety, the inspection report is more accurate and reduces maintenance times. To reduce time-to-repair and revenue losses associated with component failures, companies are creating predictive maintenance solutions. Australian company Ping has developed an aero-acoustic detector that uses sound to detect damage over blades by analyzing airflow and giving quick alerts once detected.
More specifically, innovations in floating offshore wind are accelerating along with the strong interest in this technology. Indeed, countries with few shallow sites, such as Japan and the US, see this as a significant opportunity. French startup Ideol is a major player in this market. In 2018, they installed their first floating demonstrator in Saint-Nazaire, successfully constructed floating wind turbines off the coast of Japan, and are currently building (2021-2022) a new floating wind farm in the Mediterranean sea.
Although the offshore wind sector has witnessed many innovative technologies, its large-scale implementation is limited by some remaining challenges. Above all, costs remain high (R&D, components, installations, etc.), large investments are needed, so economies of scale are still limited. Secondly, the lack of onshore electrical infrastructure to support offshore wind farms is a technical difficulty that needs to be addressed. HVDC (high-voltage direct current) electric transmission seems to be a good solution for long-distance transmission, as it reduces losses and cable costs. The cost of transmission, on the other hand, remains pretty high. A few demonstrations of this new technology are on-going in the North Sea. Finally, there are several environmental issues associated with the installation of the windfarms. A few examples are the inference of fishing sites and the collisions with birds and disturbance of nesting grounds. Orsted, the Danish group that has led the growth of wind at sea, is now desperately trying to find a way to look after a colony of kittiwake seabirds that is holding up a $10bn project off eastern England.
To conclude, offshore wind energy is an attractive and increasingly mature market. From a sector once driven by considerable government incentives and support, we are moving towards a market-driven industry. Innovations such as LiDAR, floating wind turbines and AI are having a game-changing impact on the sector.
2 Key Figures
88 Offshore wind startups
registered by Crunchbase
Market size expected to reach $59.9bn by 2025
The market size of offshore wind energy is expected to reach $59.9bn by 2025, a CAGR
3 startups to draw inspiration from
This week, we identified three startups that we can draw inspiration from: 2-B Energy, Ideol, and ONYX InSight.
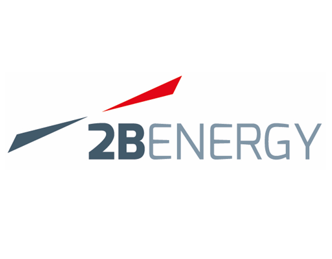
2-B Energy
2-B Energy is a startup specialised in developing wind turbine generators and power plants. It sells a turnkey solution, including the fully-integratd towers and foundation design. 2-B Energy focuses on driving costs down to make offshore wind more sustainable.
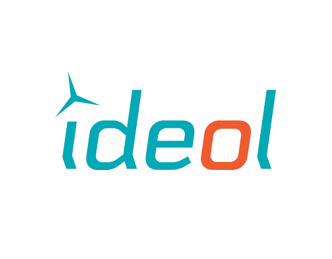
Ideol
Ideol is a leading startiup in floating foundations for offshore wind. It contributes to all stages of floating wind projects, from conception to installation. Their core product is their Damping Pool® floating technology.
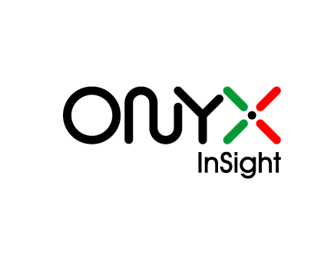
ONYX InSight
ONYX InSight provides a condition-monitoring and predictive maintenance software in the wind industry. It collects, monitors and analyses data in real time, to predict faults and make tailored maintenance recommendations.
123Fab #31
1 topic, 2 key figures, 3 startups to draw inspiration from
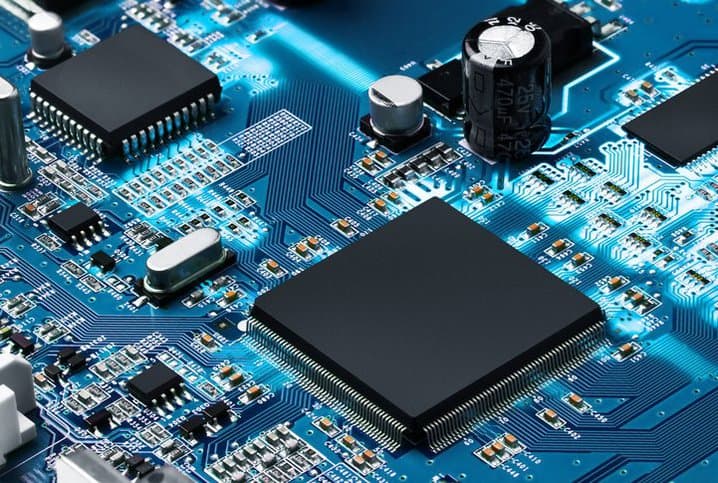
“Every 18 months, we see a doubling in the number of consumer products claiming to contain nanomaterials in Europe”, said Steffen Foss Hansen, associate professor at the Technical University of Denmark and co-founder of The Nanodatabase.
Nanomanufacturing is the production of improved and nanoscale materials, structures, devices, and systems. There are two approaches to nanomanufacturing. The first is the top-down approach, which consists of reducing large pieces of materials all the way down to the nanoscale, and the second is the bottom-up approach, which consists of creating products by building them up from atomic- and molecular-scale components. The term nanofabrication is often used instead of nanomanufacturing, but they are two very different concepts that differ in their economic dimension. While nanofabrication refers to researching and testing the feasibility of developing nano-scale materials and processes, mainly at the laboratory level, nanomanufacturing refers to the industrial-scale manufacture of nanotechnology-based objects, with emphasis on low cost and reliability.
If nanomanufacturing was mainly used for electronics (aiming to put the power of all of today’s present computers in the palm of your hand), applications in other industries are now emerging. In the solar energy sector, for instance, installation costs have been reduced by manufacturing flexible solar cell rolls instead of rigid crystalline panels. Batteries made from nanomaterials can be recharged much faster than conventional batteries. It also contributes to improving air quality thanks to a better performance of catalysts used to transform vapors escaping from cars or industrial plants into harmless gasses. Finally, several applications have been developed for the construction industry to improve the durability and enhanced performance of construction components (e.g., carbon nanotubes for better durability and crack prevention of cement), energy efficiency and safety of the buildings, facilitating the ease of maintenance and to provide increased living comfort.
All these applications of nanomanufacturing rely on a growing number of processes, mentioned above.
The top-down approach:
- Nanoimprint lithography: a process for creating nanoscale features by “stamping” or “printing” them onto a surface. A great example of such a process is Canon Nanotechnologies, the market and technology leader for high-resolution, low cost-of-ownership nanoimprint lithography systems and solutions for the semiconductor industry. Their innovative Jet and Flash Imprint Lithography technology creates the extremely small features required in today’s state-of-the-art semiconductor memory devices.
However, the process requires a lot of energy, uses chemicals (sometimes very toxic), and produces waste. Often, the results are quite unique and not easily replicable. This is why bottom-up processes are increasingly being used:
The bottom-up approach:
- Chemical vapor deposition: a process in which chemicals react to produce very pure, high-performance films. The US-based startup Grolltrex is a manufacturer of single-layer graphene sheets that uses a patented transfer and processing chemical vapor deposition method. This method allows Grolltrex to create high-performance graphene products, in addition to offering them at lower costs.
- Dip pen lithography: a process in which the tip of an atomic force microscope is “dipped” into a chemical fluid and then used to “write” on a surface, like an old-fashioned ink pen onto paper.
- Self-assembly: a process in which a group of components is assembled to form an ordered structure without outside direction. Scientists continue to explore this concept, which has become especially important in the field of nanotechnology. Indeed, as miniaturization reaches the nanoscale, conventional manufacturing technologies are failing because it has not yet been possible to build machinery that assembles nanoscale components into functional devices.
Although the applications of nanomanufacturing are wide and promising (in electronics, healthcare, energy, environmental issues, etc.), major challenges explain the slow transition from lab demonstration to industrial-scale manufacturing. The main obstacles include:
- Developing production techniques that are economically viable
- Controlling the precision of the assembly of nanostructures
- Testing the reliability and establishing methods for defect control. Currently, defect control in the semiconductor industry is non-selective and takes 20-25% of the total manufacturing time. Removal of defects for the nano-scale systems is projected to take up much more time because it requires selective and careful removal of impurities.
- Maintaining the nano-scale properties and quality of nano-system in high-rate and high-volume production.
- Assessing the environmental and social impacts, as the emergence of nanotechnology has led to the pollution of trillions of minuscule plastic particles in the oceans, waterways, and even in our bodies. High-tech workers are exposed to unusual solvents and rare earth materials that have raised safety concerns.
To scale, not only does nanomanufacturing require more time and investment to scale, but the health and environmental issues linked to the production of new nano-elements need to be addressed quickly. Nanomanufacturing would, if these issues are solved, play a key role in the innovations of many industries.
2 Key Figures
184 Nanomanufacturing startups
registered by Pitchbook
Market size expected to reach $122bn by 2025
The market size of nanoproducts is expected to reach $122bn by 2025, a CAGR of 14.3% from 2020.
3 startups to draw inspiration from
This week, we identified three startups that we can draw inspiration from: NanoMech, Nano-C, and Advano.
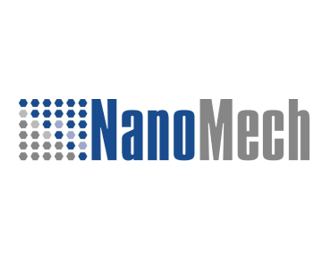
NanoMech
NanoMech manufactures lubricants, coatings, and cutting tools with their proprietary nanoscale additives that increase production rates and part quality while reducing setup times and costs.
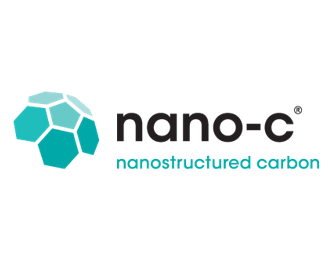
Nano-C
Nano-C develops nanostructured carbons for use in high-value energy and electronics applications. It develops nanostructured carbons for use in high-value energy and electronics applications.
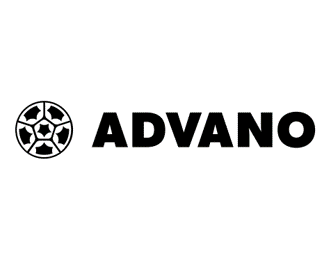
ADVANO
ADVANO combines nanotechnology with fundamental chemical engineering principles to accelerate the renewable energy revolution. Its silicon nanoparticles increase the energy density lithium-ion batteries 30-40% without sacrificing battery life or increasing the battery cost.
123Fab #30
1 topic, 2 key figures, 3 startups to draw inspiration from
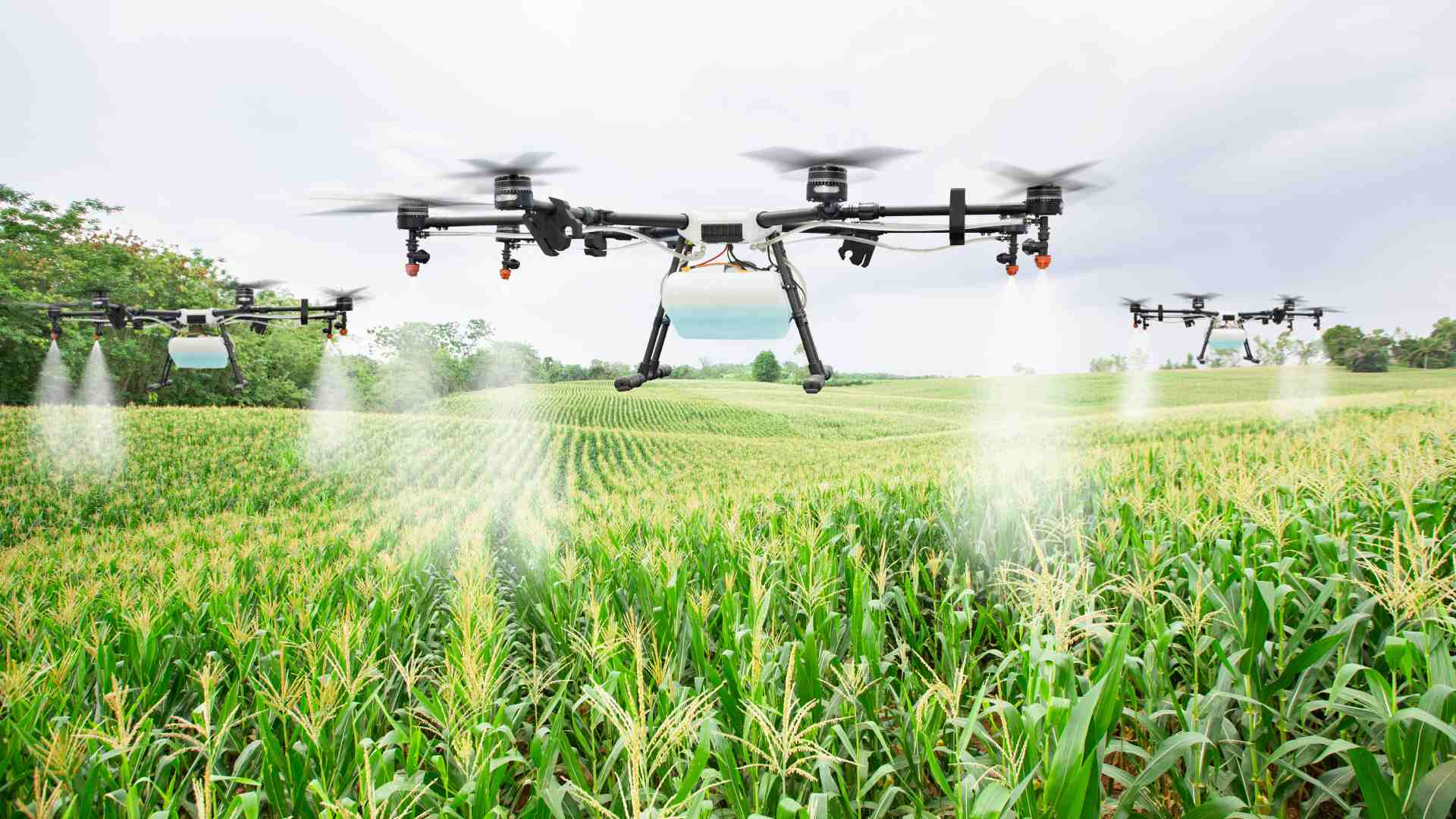
This month, the South African AgriTech startup Aerobotics raised $137M Series B for its precision agriculture platform. Using a combination of satellite imagery, drones and AI-based analytics, the startup helps farmers monitor their crops, reduce their carbon footprint and increase their overall crop yield. This funding round is just one example of the rise of precision agriculture as a promising answer to the global food crisis. Indeed, the AgriFood Tech Investment Review report indicates that investment in digital technologies accounted for 41% of the deal activity in 2019.
Precision agriculture can be defined as an approach to farm management that uses information technology (IT) to ensure that crops and soils receive exactly what they need for optimum health and productivity. The data used in precision agriculture can be collected 2 ways:
- Aerial imagery – drones, planes and satellites help to create bird-eye views of the cultivated area, which can be used to analyze a number of parameters such as the amount of water in the soil or the health and maturity of a crop. Normalized Difference Vegetation Index (NDVI) imagery is a method of measuring crop health based on the greenness of a plant.
- Soil sensors – these are used to measure the most essential parameters and chemical properties of the soil. They can be electromagnetic, electrochemical, mechanical, etc. Electrochemical sensors provide information on nutrient availability and pH in the soil, allowing crop stress and diseases to be detected 3-4 weeks in advance.
Multiple technologies have been developed in recent years for different applications: farm planning, field mapping, soil sampling, tractor guidance, crop scouting, yield mapping, etc. By moving from homogenous to individual processing, these technologies have impacted the entire agricultural value chain – from input supply to the end customer – and improved crop yields while achieving sustainability goals (limited resource use and environmental degradation). Among the technologies that have been developed, 5 main ones can be identified:
- Remote sensing technology – these technologies use remotely sensed data to measure the most essential parameters on a farm.
- Variable rate technology (VRT) systems – they use the data collected to automate the amount of input (seeds, fertilizers, pesticides, water) applicable within defined farming areas. These technologies can be used for seeding crop fields, spraying pesticides, applying fertilizers, spreading manure, etc. Irrigation systems are the most common control systems. They allow the exact amount of water to be distributed. Startup CropX has developed an analytics software that integrates with irrigation systems to help increase crop yields.
- Satellite positioning systems – global positioning systems (GPS) enable to calculate precise locations and positions. These systems are used for navigation but also for geo-referencing information. For instance, farmers use GPS to collect geo-referenced soil samples to check nutrients, pH levels and other data to make profitable decisions.
- Equipment guidance and automated steering systems – they automate farmers’ slow, repetitive and tedious tasks, such as harvesting crops or blowing seeds. Autonomous machines are slowly appearing in the industry, using computer vision to distribute fertilizers accurately – studies show that fertilizer use can be reduced by up to 80%.
- Geo-mapping – it is a technology used to create maps of various soil and crop conditions.
In recent years, large agricultural players have joined forces with startups to shape the future of the agricultural industry. ADAMA, one of the world’s leading crop protection companies, recently announced a partnership with startup Taranis, to develop an end-to-end precision agriculture solution.
Although precision agriculture is a golden opportunity for global food security and crop yields, some challenges still need to be addressed. One of the first obstacles to its adoption is the high investment costs. Another is that most solutions have been designed for large, homogenous farms and are therefore not suitable for small, diversified farms. In addition, as precision agriculture has only recently taken off, there is still is a lack of experience in the use of drones, robots, and other precision agriculture tools, as well as a lack of connectivity between all the different devices and software (but should fame over time). Last but not least, the complexity of managing data privacy and cybersecurity is problematic (most of the cyber threats faced by precision agriculture are consistent with those in other connected industries: data theft, theft of resources, reputation loss, destruction of equipment, or gaining an improper financial advantage over a competitor).
To conclude, precision agriculture is undergoing a rapid transformation. The use of GPS guidance, aerial mapping, robotics, and drones is pushing towards sustainable agricultural practices that can have a positive impact on social, environmental, and economic aspects, but it remains a challenging opportunity. While not all companies will succeed in the transition, those that do will shape tomorrow’s sustainable food supply.
2 Key Figures
210 Precision agriculture startups
registered by Crunchbase
Market size expected to reach $11.1bn by 2025
The market size is expected to reach $11.1bn by 2025, rising at a CAGR of 13.9%.
3 startups to draw inspiration from
This week, we identified three startups that we can draw inspiration from: GramworkX, Karnott and Arable.
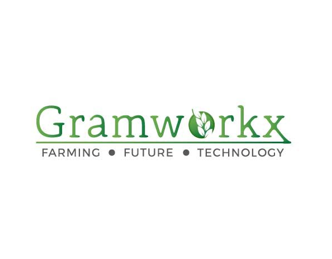
GramworkX
GramworkX has developed an IOT and AI enabled smart farm resource management tool, which helps the farmers guide, optimize and monitor utilization of water. The technology includes a unique machine learning algorithm, which provides micro-climatic condition predictions for the farmer to take accurate and proactive decisions.
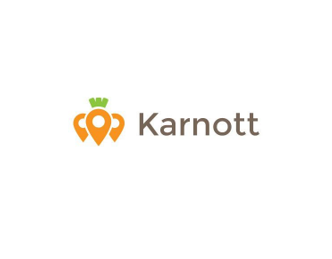
Karnott
Karnott offers a tracking software coupled with a connected device that tracks agricultural equipment. Karnott places an automatic, autonomous, mobile, real-time device in tractors, trailers, or seeders to collect data, and then their software automatically calculates, analyzes, and archives it.
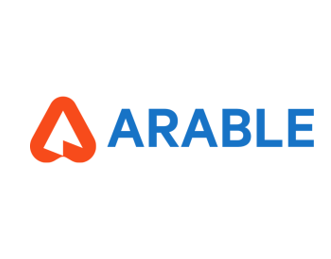
Arable
Arable is an agricultural data and analytics company that offers the world’s first IoT-enabled irrigation management tool, weather station, and crop monitor in one, the Arable Mark. Reliable data-driven decision-making saves customers time and money, reducing risk while preserving natural resources.